Uncover New Drug Targets Through Cell Metabolism
eBook
Published: February 15, 2023
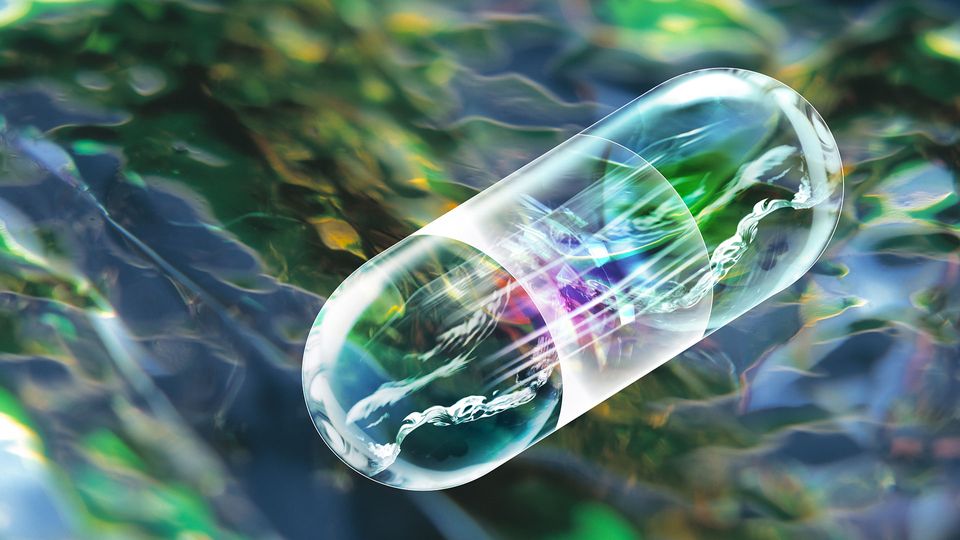
Advanced technologies such as live-cell metabolic analysis can help researchers uncover key vulnerabilities in disease-associated pathways. Moreover, it can help identify and validate potential drug targets effectively and support quick decision-making during the drug discovery process.
This eBook presents examples of promising metabolic targets in a variety of therapeutic areas that can be incorporated into your target identification workflows.
Download this eBook to discover more about:
- Metabolic reprogramming in various diseases
- The latest advancements in metabolism for target identification
- Data examples showing our live-cell metabolic analysis provides unique insights to assist target identification research
Uncover New Drug Targets
Through Cell Metabolism
Drug Target eBook
Cellular Metabolism
A New Avenue in Drug Discovery Topics
Drug target identification is a key step in the discovery and
development of safe and effective therapies. Often thought of as
simply providing ‘housekeeping’ functions, energy metabolism
is now emerging as a critical contributor to many cellular
functions. Dysfunctional metabolism is also associated with a
growing number of different disease states. Therefore, looking
at the genes, proteins, and pathways that modulate energy
metabolism is a promising new avenue for developing novel
therapeutic strategies for a broad range of diseases.
This handy reference eBook provides examples of metabolic
targets impacting key disease areas including:
- Cancer
- Immuno-oncology/Immunotherapy
- Neurodegenerative diseases
- Diabetes, Cardiovascular and Acquired Metabolic Disorders
We hope that this eBook will inspire you to look at your
therapeutic strategy in a new way, through the lens of energy
metabolism. To learn how you can incorporate Agilent solutions
for measuring energy metabolism into your target identification
workflows, see more here:
www.agilent.com/chem/drugdiscovery-cellmetabolism
3
Cancer
Altered energy metabolism is now recognized as a hallmark of cancer1
. Identification of targets affecting metabolic
pathways, therefore, provides critical insights into potential effective cancer therapies.
4
Target: Oncogenes, associated molecular
targets and metabolic pathways
Oncogenes are responsible for rewiring cancer cell
energy metabolism towards biomass accumulation and
glycolytic energy production, rather than the mitochondrial
energy production that occurs in nonproliferating cells.
Intermediates in oncogene pathways (including genes,
proteins, and enzymes) represent promising targets for
cancer therapies.
Target: Nutrient transport and utilization
To support biomass accumulation, cancer cells
increase substrate and nutrient uptake from the tumor
microenvironment. Potential metabolic targets may
focus on inhibiting or activating genes, proteins, and
pathways associated with substrate and nutrient
transport or utilization.
Phenogram: Simultaneous measurement of
oxidative phosphorylation and glycolysis
40
OCR (pmol/min)
OCR (pmol/min)
ECAR (mpH/min) ECAR (mpH/min)
glycolytic glycolytic
Oxidative
Chemoresistance
isogenic
counterpart
Chemosensitive
Oxidative 150 50
40
100
30
30
50
10
0 0
0 20 40 60 80 0 10 20 30
Energetic Energetic
A2780 PEO1
C200
Learn more
Determine substrate dependency of cancer Learn more
cell models
Time (min)
Inhibitor Oligo FCCP Rot/AA
0 24 48 72 96 120
OCR (pmol/min/1,000 Cells)
1
2
3
4
5
6
7
8
9
10
0
Cancer
Significant advances have been made in defining druggable targets in cancer cells, with metabolic intermediates being highlighted as
key targets in cancer drug discovery.
PEO4
A549 Mitochondrial Respiration
Control
Etomoxir
(LCF As)
UX5099
(Glucose/Pyruvate)
BPTES
(Glutamine)
Adapted from Dar, S., et al. Bioenergetic Adaptations in Chemoresistant Ovarian Cancer Cells.
Sci Rep. 2017. 7 (1): 8760.
5
Table 1 lists several promising metabolic targets for cancer therapies. The stage of development and effectiveness of these metabolic
targets range from preclinical to clinical and postclinical and represent areas of opportunity for drug target identification and validation.
Target ID Pathway/Function Description References
GLUT1 Glycolysis, glucose transport Glucose transporter 2
xCT Glutamine, glutamate metabolism - cystine
acquisition for GSH synthesis Cystine-glutamate antiporter encoded by SLC7A11 gene 3
MCT1 Lactate metabolism Monocarboxylate transporter encoded by SLC16A1 gene 4-6
GAPDH Glycolysis Glycolytic enzyme 7
LDHA Glycolysis, NAD/NADH balance Glycolytic enzyme, catalyses pyruvate-lactate conversion 8-13
GLS Glutamine metabolism Amidohydrolase enzyme, catalyzes
glutamine-glutamate conversion 14-18
CPT1 Long-chain fatty acid
transport/oxidation
Mitochondrial enzyme, catalyzes transfer of an acyl group
of a long chain fatty acyl-CoA from coenzyme A to L-carnitine 19
PDK1 Pyruvate metabolism/oxidation Protein kinase that regulates pyruvate dehydrogenase (PDH) activity 20
MGLL Fatty acid synthesis Monoacylglycerol lipase, catalyze hydrolysis of monoacilglycerides into
glycerol and long-chain fatty acids 21
HIF1 Regulates the hypoxic response Hypoxia-inducible transcription factor 22
mTOR Regulates cell proliferation, transcription,
translation, and autophagy Kinase encoded by MTOR gene 23-24
Table 1. Examples of promising metabolic targets for cancer therapies.
Therapeutic targeting of metabolic synthetic lethality in cancer research
“The rapid proliferation of cancer cells requires simply more energy, ATP, and macromolecules.
Therefore cells have to rewire their metabolic network to meet such demands. That opens many
potential opportunities for targeting tumor metabolism in cancer therapy.”
– Yuting Sun, PhD
Senior Research Scientist, MD Anderson Cancer Center
View eSeminar
Cancer
6
Immuno-oncology / Immunotherapy
Modulating the activity of immune cells for the treatment of a variety of pathologies such as cancer or autoimmune
diseases is one of the fasting growing areas in drug discovery. Metabolic reprogramming plays a key role in the
immediate response of the immune cell, as well as directing cell fate. Different immune cell types have unique metabolic
requirements, to support energetic and biosynthetic demands.
*
* *
*
7
The balance of nutrients and metabolites in the tumor microenvironment can significantly affect metabolic programs, altering
immune cell response. Targeting critical metabolic pathways is emerging as a promising strategy to regulate immune function and
has been shown to strongly influence the efficacy of immune checkpoint as well as adoptive CAR-T therapies.
Monitor real-time immune cells response to checkpoint blockade
Bezafibrate enhances the effectiveness of PD-L1 blockade in antitumor immunity by activating mitochondrial respiration of killer T cells to promote survival and proliferation
and improve effector function.
Adapted from Chowdhury, P. S. et al. PPAR-Induced Fatty Acid Oxidation in T Cells Increases the Number of Tumor-Reactive CD8(+) T Cells and Facilitates Anti-PD-1 Therapy.
Cancer Immunol Res. 2018. 6 (11): 1375-1387.
Time (min)
FCCP Rotenone/
Antimycin A
Oligomycin
OCR (pmol/min)
200
150
100
50
0
0 20 40 60 80 100 120
anti-PD-L1
Bezafibrate
ECAR(mpH/min)
OCR (pmol/min)
Spare respiratory capacity (pmol/min)
120
80
40
0
+
– –
– +
+
0
0 30 35 40 45
50
60
70
80
Learn more
Immuno-oncology / Immunotherapy
Ctrl IgG anti-PD-L1 anti-PD-L1 + bezafibrate
8
Monitor immune cell fitness and function
Core metabolic pathways dictate potency and persistence in adoptive T cell therapy
“There’s a huge interest in how energetic metabolism really regulates the core immune
function. Much of that work has really come from our understanding and study of T cells:
how metabolism regulates T cell differentiation, and T cell effector function.”
– John Connolly, PhD
Chief Scientific Officer, Tessa Therapeutics Ltd
Research Director, Institute of Molecular and Cellular Biology (IMCB)
Cellular bioenergetics plays a critical role in determination of fate of engineered immune cells.
Cell Fate
Tuning
CAR
Engineering
CAR
Engineering
CD28, TCR 4-1BB TCR
Immune Memory
Persistence
Central Memory
Oxidative Phosphorylation
Tumor Killing
Effector memory
Aerobic glycolysis
T cell
Cell Fate
Tuning
Activated
Vehicle
anti-CD3/CD28
Baseline
Fit
Oligo FCCA R/A
Ovarian Cancer
Relative OCR (%)
Time (min)
Ascites (%)
Fitness
Exhausted
200
150
100
50
30 60 90 120
0
0
Real-time Activation
Injection of anti-CD3 and anti-CD28 activator
Fate & Persistence Fitness
Learn more
View eSeminar
Immuno-oncology / Immunotherapy
Time (min)
Time (min)
Oligo FCCA R/A
Ovarian Cancer
ECAR (mpH/min)
Relative OCR (%)
Vehicle
anti-CD3/CD28
05
0
10
20
200 30
150
100
50
0
0 30 60 90 120
0 1001 50
Ascites (%)
Fit
Exhausted
9
Mitochondrial dysfunction has been implicated as the most common cause of neurodegenerative disease, which is primarily
linked to dysfunctional respiration (OXPHOS), altered homeostasis of metabolic intermediates and trigger of cell death
mechanisms25. Identification of specific proteins affecting mitochondrial metabolic function provides insight into potential
drug targets for neurodegenerative disease.
Neurodegenerative Diseases
10
Mitochondria are cellular powerhouses responsible for the production of energy (ATP) and the regulation of several other processes that
are critical to cellular homeostasis. Neuronal cells have high energy demands and limited regeneration capabilities; consequently, these
cells are highly dependent on mitochondrial function for survival. Indeed, there is increasing evidence that mitochondrial proteins and
mitochondrial dysfunction are responsible for the onset of neurodegenerative diseases, including Alzheimer’s, Parkinson’s, Huntington’s,
and amyotrophic lateral sclerosis (ALS).
Target: Mitochondrial proteins
and intermediates in
mitochondrial dysfunction
Studies measuring metabolic pathways
activity and intermediates indicate
that mitochondrial dysfunction is the
primary cause of neurodegenerative
disease. Metabolic targets and
key driving factors are attributed
to inherited mutations (mtDNA
mutations), mitochondrial membrane
permeability and potential disruption,
disrupted mitochondrial fusion or
fission, impaired protein and ion
homeostasis, reactive oxygen species
or accumulation of toxic aggregates,
and dysfunctional mitophagy.
Neurodegenerative Diseases
Mitochondrial Spare Respiratory Capacity provides
a strong indicator of neurodegenerative disease
Adapted from Theurey, P., et al. Systems biology identifies preserved integrity but impaired metabolism of
mitochondria due to a glycolytic defect in Alzheimer's disease neurons. Aging Cell. 2019. 18 (3): e12924.
Learn more
OCR
(pmol O/min/µg protein)
OCR (pmol O2/min/µg protein)
Time (min)
Non-mitochondrial OCR
Basal
Maximal OCR
Spare
capacity
Oligo FCCP Anti A
ATP
synthesis
H leak
Oligo FCCP Anti A
Basal
WT
WT
**
***
TgAD
TgAD
H+ lcak ATP
synthesis
Maximal
OCR
Non-mito.
OCR
Spare
capacity
0
4
8
12
16
20
25
20
15
10
5
20 40 60
0
0
**
11
Table 2 lists some mitochondrial proteins that have been studied as potential targets for neurodegenerative disorders. Mitochondrial
proteins represent a relatively new target for drug development compared to other drug targets in neurodegenerative diseases. Although
few therapies targeting mitochondrial proteins have reached the stage of clinical trials, they represent a promising area of opportunity for
novel targets.
Target ID Pathway/Function Description Diseases References
CypD Mitochondrial function/health Peptidyl prolyl isomerase F, associates with the inner
mitochondrial membrane during the mitochondrial membrane
permeability transition (mPTP formation)
Alzheimer's, Parkinson's,
amyotrophic lateral
sclerosis (ALS)
26–28
PINK1 PInk1/Parkin pathway, mitophagy Mitochondria-specific kinase PTEN Induced Kinase 1 Parkinson's 29
LRRK2 Cytoplasmic GTPase and kinase activites Member of the leucine-rich repeat kinase family Parkinson's 30–32
Drp-1 Mitophagy GTPase that regulates mitochondrial fission Parkinson's 33–34
DJ-1 Cellular oxidative stress response Redox-sensitive chaperone/sensor for oxidative stress,
encoded by PARK7 gene
Parkinson's 35
ABAD Oxidation of isoleucine, branched-chain fatty acids,
xenobiotics, sex hormones, and neuroactive steroids
Mitochondrial enzyme encoded by the HSD17B10 gene Alzheimer's 36–39
MPC Pyruvate oxidation Mitochondrial pyruvate carrier Neurodegeneation due to
excitotoxic injury
40
SOD1 Antioxidant enzyme, detoxification of ROS in cells Superoxide dysmutase isoform present in the
cytoplasm and mitochondrial intermembrane space,
encoded by SOD1
Amyotrophic lateral
sclerosis (ALS)
41–42
Table 2. Examples of promising metabolic targets for neurodegenerative disorders.
Mitochondrial pyruvate metabolism controls neuronal function and excitotoxic death
“There’s quite a bit of history about the limitations that we have had over the years, basically up until the
development of high-throughput respirometry, in what we could learn, and certainly the speed in which we could
gather data.”
–Anne N. Murphy
– Ajit S. Divakaruni, PhD
Asst. Professor of Molecular and Medical Pharmacology,
University of California, Los Angeles
– Anne N. Murphy, PhD
Professor of Pharmacology,
University of California, San Diego
Neurodegenerative Diseases
View eSeminar
Environmental factors relating to excess nutrients and stress are key drivers of obesity, leading to further complications,
including type 2 diabetes and cardiovascular disease. Modulation of metabolic targets to restore the balance of energy
homeostasis to “normal” represents a promising therapeutic strategy for reversing or preventing these damaging syndromes.
12
Diabetes, Cardiovascular,
and Other Acquired Metabolic Disorders
13
Different tissues are designed to use different nutrients to meet their energy demands. Excessive nutrient and substrate availability,
or long-term exposure to the wrong balance of substrates have a cascade of effects, including increased adipose tissue, inflammation,
and insulin resistance. Identifying those proteins active in energy substrate uptake and utilization, including metabolic pathways and
intermediates, are rich sources of potential drug targets.
Target: Lipid versus glucose metabolism, and insulin resistance
There is a negative feedback between lipid metabolism and glucose metabolism as an energy source. The energy balance
between fatty acid oxidation and glucose oxidation (including reducing mitochondrial uncoupling) is affected by the activity of
proteins and enzymes involved in substrate uptake and utilization.
Diabetes, Cardiovascular, and Other Acquired Metabolic Disorders
Natural products that expand and induce Brown adipose tissue using innovative
Stem Cell Metabolism Assays.
– Shahzad Ali, PhD,
Senior Research Scientist, Plasticell Limited
Illustrative Discovery Workflow
Step 1: Validate Phenotype of in vitro Model of Brown
Adipose Tissue (BAT)
Step 2: Screen for Metabolic Activators of BAT Step 3: Select and Re-characterise Leads for Mechanism
of Action and Development
Agilent MitoXpress Xtra
Oxygen Consumption
Assay: Identify Hits
increasing basal OXPHOS
Agilent Seahorse XF
Cell Mito Stress Test:
Higher metabolic
activity and proton leak,
characteristic of BAT
Example Hit compound with increased basal
and spare respiratory capacity
View eSeminar
OCR (pmol/min)
Time (min)
1µM
Antimycin
0.2%
DMSO
extract 8
OCR
0 20 40 60 80 100
100
90
80
70
60
50
40
30
20
10
00
0
2
4
6
8
10
12
800
600
400
200
Time (min)
ADSC-d BAI
Antimycin A
Rotenone
FCCP
Oligomycin
ADSC-d WAT
OCR (pmol/min)
0
0 50 100 150 200
14
Table 3 lists proteins that have been studied previously as potential targets for metabolic disorders relating to obesity, diabetes, and
cardiovascular disease.
Target ID Pathway/Function Description References
PPARα Controls expression of (hepatic) genes involved in fatty acid uptake and intracellular
transport, fatty acid oxidation, lipogenesis, ketogenesis, and lipoprotein/cholesterol
metabolism (in adipocytes)
Ligand activated nuclear receptor/transcription factor 43
PPARγ Regulation of ADD, function, insulin sensitivity, lipogenesis, lipid storage,
and glucose metabolism
Ligand activated nuclear receptor/transcription factor 44
AMPK AMPK activation results in stimulation of hepatic / skeletal fatty acid
oxidation, ketogenesis, and glucose uptake, inhibition of FA synthesis, lipogenesis,
and modulation of insulin secretion
ADP-activated protein kinase, a key enzyme in cellular
energy homeostasis
45
GLUT4 Glycolysis/glucose oxidation Insulin-regulated glucose transporter 46, 47
CPT1 Long chain fatty acid transport/oxidation Mitochondrial enzyme, catalyzes transfer of an acyl group of a
long chain fatty acyl-CoA from coenzyme A to L-carnitine
45
MPC Pyruvate oxidation Mitochondrial pyruvate carrier, therapeutic target for modulating
energy balance and the metabolic profile
48
UCPs ETC/OXPHOS Family of mitochondrial proteins present mainly in brown adipose
tissue, uncouples ETC from OXPHOS
49
Table 3. Examples of promising metabolic targets for diabetes, cardiovascular, and other aquired metabolic disorders.
Impaired mitochondrial ß-oxidation in cardiomyocytes: role of the cardiac renin-angiotensin system
and miR-208
“The pathogenic mechanisms of diabetic cardiomyopathy are complex. What is central in the
diabetic heart are changes in it’s energy substrate utilization and metabolism.”
– Margriet Ouwens Ph.D.
German Diabetes Center,
DDZ Institute for Clinical Biochemistry and Pathobiochemistry, Düsseldorf
Diabetes, Cardiovascular, and Other Acquired Metabolic Disorders
View eSeminar
15
Explore how you can incorporate Agilent solutions for measuring energy metabolism into your drug
discovery workflows
Discover Agilent Cell Analysis Publications
Visit the eSeminars web page to view past webinars on-demand
Learn how to run our assays
Meet with an Expert
Useful Resources
16
Citations
1. Hanahan, D., & Weinberg, RA. (2011) Hallmarks of Cancer. The Next
Generation. Cell. 144(5), 646-674.
2. Chan, D. A. et al. (2011) Targeting GLUT1 and the Warburg effect in renal cell
carcinoma by chemical synthetic lethally. Sci. Transl. Med. 3, 94ra70.
3. Timmerman, L. A. et al. (2013) Glutamine sensitivity analysis identifies the
xCT antiporter as a common triple-negative breast tumor therapeutic target.
Cancer Cell. 24, 450–465.
4. Polanski, R. et al. (2014) Activity of the monocarboxylate transporter 1
inhibitor AZD3965 in small cell lung cancer. Clin. Cancer Res. 20, 926-937.
5. Birsoy, K et al. (2013) MCT1-mediated transport of a toxic molecule is an
effective strategy for targeting glycolytic tumors. Nature Genet. 45, 104–108.
6. Sonveaux, P. et al. (2008) Targeting lactate-fueled respiration selectively kills
hypoxic tumor cells in mice. J. Clin. Invest. 118, 3930–3942.
7. Ganapathy-Kanniappan, S. et al. (2013) Anticancer efficacy of the metabolic
blocker 3-bromopyruvate: specific molecular targeting. Anticancer Res. 33,
13–20
8. Boudreau, A. et al. (2016) Metabolic plasticity underpins innate and acquired
resistance to LDHA inhibition. Nat. Chem. Biol. 12, 779–786.
9. Cui, W. et al. (2016) Discovery of 2-((3-cyanopyridin-2-yl) thio) acetamides as
human lactate dehydrogenase A inhibitors to reduce the growth of MG-63
osteosarcoma cells: virtual screening and biological validation. Bloorg. Med.
Chem. Lett. 26, 3984–3987.
10. Billiard, J. et al. (2013) Quinoline 3-sulfonamides inhibit lactate
dehydrogenase A and reverse aerobic glycolysis in cancer cells. Cancer
Metab. 1, 1-19.
11. Rai, G. et al. (2017) Discovery and optimization of potent, cell active
pyrazole-based inhibitors of lactate dehydrogenase (LDH). J. Med. Chem. 55,
3285–3306.
12. Ward, R.A. et al. (2012) Design and synthesis of novel lactate dehydrogenase
A inhibitors by fragment-based lead generation.J. Med. Chem. 55,
3285–3306.
13. Le, A. et al. (2010) Inhibition of lactate dehydrogenase A induces oxidative
stress and inhibits tumor progression. Proc. Natl. Acad. Sci. 107, 2037–2042.
14. Xiang, Y. et al. (2015) Targeted inhibition of tumor-specific glutaminase
diminishes cell-autonomous tumorigenesis. J. Clin. Invest. 125, 2293–2306.
15. Shroff, E. H. et al. (2015) MYC oncogene overexpression drives renal cell
carcinoma in a mouse model through glutamine metabolism. Proc. Natl.
Acad. Sci. 112, 6539–6544.
16. Gross, M.I. et al. (2014) Antitumor activity of the glutaminase inhibitor CB839 in triple-negative breast cancer. Mol. Cancer Ther. 13, 890–901.
17. Wang, J. B. et al. (2010) Targeting mitochondrial glutaminase activity inhibits
oncogenic transformation. Cancer Cell 18, 207–219.
18. Seltzer, M. J. et al. (2010) Inhibition of glutaminase preferentially slows
growth of glioma cells with mutant IDH1. Cancer Res. 70, 8981–8987.
19. Pike, L. S. et al. (2011) Inhibition of fatty acid oxidation by etomoxir impairs
NADPH production and increases reactive oxygen species resulting in ATP
depletion and cell death in human glioblastoma cells. Biochim. Biophys. Acta
1807, 726–734.
20. Michelakis, E. D., Webster, L. & Mackey, J. R. (2008) Dichloroacetate (DCA) as
a potential metabolic-targeting therapy for cancer Br. J. Cancer 99, 989–994.
21. Nomura, D. K. et al. (2010) Monoacylglycerol lipase regulates a fatty acid
network that promotes cancer pathogenesis Cell 140, 49–61.
22. Wilson, W. R. & Hay, M. P. (2011) Targeting hypoxia in cancer therapy. Nature
Rev. Cancer 11, 393–410.
23. Sabatini, D. M. (2006) mTOR and cancer, insights into a complex relationship.
Nature rev. Cancer 6, 729–734.
24. Benjamin, D. et al. (2011) Rapamycin passes the torch a new generation of
mTOR inhibitors. Nature Rev. Drug Discov. 10, 868–880.
25. Lee, J. (2016) Mitochondrial drug targets in neurodegenerative diseases.
Bioorganic & Medical Chemistry Letters. 26, 714-720.
17
26. Guo, H. X. et al. (2005) Novel cyclophilin D inhibitors derived from
quinoxalineexhibit highly inhibitory activity against rat mitochondrial swelling
and Ca2+ uptake/release Acta Pharmacol. Sin. 26, 1201–1211.
27. Valasani, K. R. et al. (2014) Design, synthesis, in silico and in vitro studies of
novel 4-methylthiazole-5-carboxylic acid derivatives as potent anti-cancer
agents Bioorg. Med. Chem. Lett. 24(18), 4580–4585.
28. Elkamhawy, A. et al. (2014) Novel quinazoline-urea analogues as modulars for
Aβ-induced mitochondral dysfunction: design, synthesis, and molecular docking
study. Med. Chem. 84, 466–475.
29. Hertz, N. T. et al. (2013) A neo-substrate that amplifies catalytic activity of
parkinson's-disease-related kinase PINK1. Cell. 154, 737–747.
30. Li, T. et al. (2015) A Novel GTP-Binding Inhibitor, FX2149, Attenuates LRRK2
Toxicity in Parkinson's Disease Models. PLoS One. 10, e0122461.
31. Li, T. & Yang, D. et al. (2014) Discovery of highly potent, selective, and brainpenetrant aminopyrazole leucine-rich repeat kinase 2 (LRRK2) small molecule
inhibitors. Med. Chem. 57, 921–936
32. Estrada, A. A. et al. (2014) Discovery of highly potent, selective, and brainpenetrant aminopyrazole leucine-rich repeat kinase 2 (LRRK2) small molecule
inhibitors. Med. Chem. 57, 921–936.
33. Qi, X. et al. (2013) A novel Drp 1 inhibitor diminishes aberrant mitochondrial
fission and neurotoxicity. Cell. Sci. 126, 789–802.
34. Lackner, L. L. & Nunnari, J. (2010) Small molecule inhibitors of mitochondrial
divisionsn tools that translate basic biological research into medicine. Chem.
Biol. 17, 578–583.
35. Kitamura, Y. et al. (2011) Neuroprotective effect of a new DJ-1-binding
compound against neurodegeneration in Parkinson's disease and stroke model
rats. Mol. Neurodegener. 6(48), 1–19.
36. Kissinger, C. R. et al. (2004) Molecular dynamics simulations of the amyloidbeta binding alcohol dehydrogenase (ABAD) enzyme. Mol. Biol. 16(21),
9511–9518.
37. Lim, Y.T. et al. (2011) Inhibitation of the mitochondrial enzyme ABAD resotres
the amyloid-β-mediated deregulation of estradiol. PLoS One.6, e28887.
38. Valaasani, K.R. et al. (2014) Identification of human ABAD inhibitors for rescuing
Aβ-mediated mitochondrial dysfunction. Curr. Alzeimer Res.11, 128-136.
39. Ayan, D., Maltais, R, & Prior, D. (2012) Identification of a 17β-hydroxysteroid
dehydrogenase type 10 steroidal inhibitor: a tool to investigate the role of type
10 in Alziheimer’s disease and prostate cancer. ChemMedChem.7, 1181-1184.
40. Divakaruni, A. S. et al. (2019) Inhibition of the nitochondrial pyruvate carrier
protects from excitotoxic neuronal death. J Cell Biol. 4, 1091-1105.
41. Kalmar, B. et al. (2008) Cellular toxicity of mutant SOD1 protein is linked to an
easily soluble, non-aggregated from in vito. Neurobiol Dis. 49, 49-56.
42. Lange, D. J. et al. (2013) Pyrimethamine decreases levels of SOD1 in leukocytes
and cerebrospinal fluids of ALS patients: a phase I plot study. Amytroph lateral
Scler Frontotemporal Desener. 14(3) 199-204.
43. Han et al. (2017) PPARs: regulators of metabolism and as therapeutic targets in
cardiovascular disease. Part I: PPAR-α Future Cardiol. 13(3), 259-278.
44. Han, L. et al. (2017) PPARs: regulators of metabolism and as therapeutic targets
in cardiovascular disease. Part Ii: PPAR- β/ δ and PPAR- γ. Future Cardiol. 13(3),
279-296.
45. Fukshima, A. et al. (2015) Myocardial Energy Substrate Metabolism in Heart
Failure: from Pathways to Therapeutic Targets. Current Pharmaceutical Design.
21,3654-3664.
46. Schreiber, I, et al. (2017) BMPs as new insulin sensitizers: enhanced glucose
uptake in mature 3T3-L1 adipocytes via PPAR gamma and GLUT4 upregulation.
Sci Rep.7 (1), 17192.
47. Bhowmik, A. & Banu, S. (2017) Therapeutic targets of type 2 diabetes: an
overview. MOJ Drug Des Develop Ther. 1(3), 00011.
48. Divakaruni, A,S. et al. (2013) Thiazolidinediones are acute, specific inhibitors of
the mitochondrial pyruvate carrier. PNAS. 110(14), 5422-5427.
49. Samudio, I. et al. (2019) Mitochondrial Uncoupling and the Warbug Effect.
Molecular basis for the Reprogrmming of Cancer Cell Metabolism. Cancer Res
69(6), 2163-2166.
Learn more
www.agilent.com/chem/drugdiscovery-cellmetabolism
Live Chat
www.agilent.com/chem/discoverXF
U.S. and Canada
1 800 227 9770
agilent_inquiries@agilent.com
Europe
UK 0800 096
Denmark 45 8830 5083
Germany 0800 180 66 78
Netherlands 0800 022 7243
Other EU 45 3136 9878
info_agilent@agilent.com
Asia Pacific
China 800 820 3278
Singapore 65 6571 0888
inquiry_lsca@agilent.com
For Research Use Only. Not for use in diagnostic procedures.
DE.6191666667
This information is subject to change without notice.
© Agilent Technologies, Inc. 2020
Published in the USA, June 18, 2020
5994-2059EN
Brought to you by
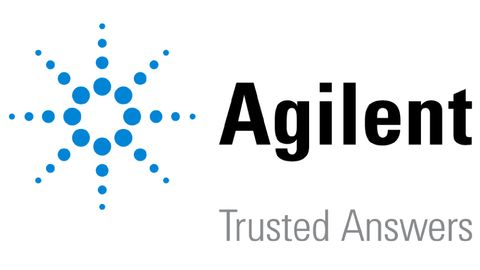
Download the eBook for FREE Now!
Information you provide will be shared with the sponsors for this content. Technology Networks or its sponsors may contact you to offer you content or products based on your interest in this topic. You may opt-out at any time.