Single Cell Analysis – Advantages, Challenges, and Applications
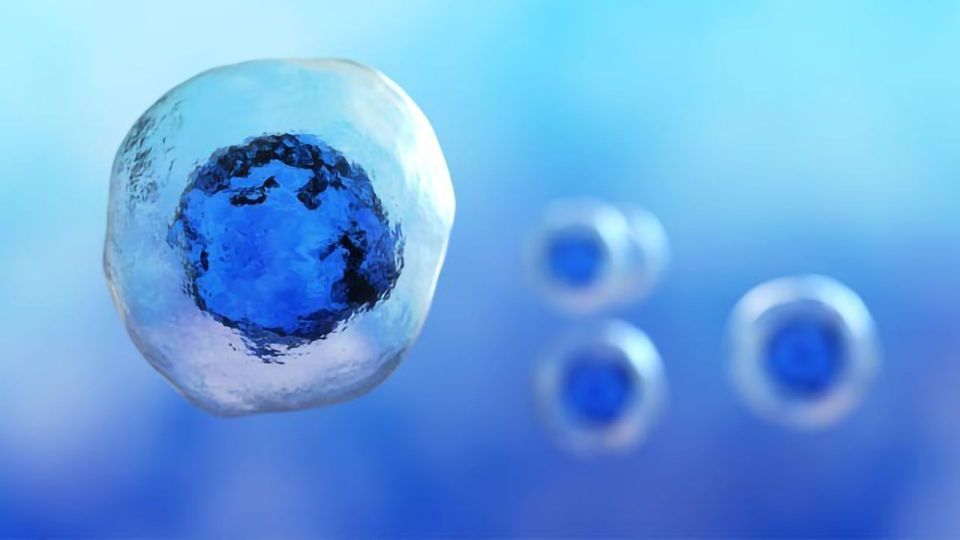
Complete the form below to unlock access to ALL audio articles.
Heterogeneity exists even within the smallest populations of cells. Being able to analyze cells on an individual level means that you gain a more comprehensive understanding of key changes "cell-by-cell" rather than those at a population level. Having information at both a single- and multi-cell level ensures you can more accurately capture critical insights.
We recently spoke with Dr Marian Rehak, VP of Research and Development at Sphere Fluidics, to learn more about the importance of single cell analysis. Marian touches on some of the common challenges encountered and highlights some of the key applications.
Laura Lansdowne (LL): Why is it so important to perform analysis at the “single-cell” level?
Marian Rehak (MR): There is a need to study cells on both multi- and single-cell levels to give scientists a balanced and all-encompassing picture. Cell-to-cell variation is a natural property of both healthy and diseased tissues or multi-cellular organisms, which presents major challenges in current drug discovery and development, diagnostics and prognostics.
Statistical analysis of whole cellular populations provides an average response across the entire population and is not fully representative of any one cell, including rare variants. So, the population average may mask the reaction of a single cell. Such heterogeneity is a major challenge when attempting to develop accurate disease models, or elucidating patient responses to specific therapies. Fundamentally, analysis of individual single cells from a heterogeneous population enables the reduction of biological noise and offers the ability to investigate and characterize rare cells.
LL: What are some of the common challenges encountered when performing single-cell analysis?
MR: One of the major challenges in single-cell analysis is the initial isolation and culture of a single cell. Cell viability is required for downstream analysis and further cell outgrowth. However, cells are not usually on their own and can be difficult to culture independently. Cells are heavily influenced by their surrounding environment and cell-to-cell interactions. Single cells that have been manipulated and isolated are no longer in their native environment, which could result in an unnatural response to the artificial environment. Additionally, the isolation process can induce unintended cell stress, altering the behavior, viability and profile of the cell.
Another limitation of single-cell analysis is processing power, as analyzing each individual cell in a population requires hundreds-of-thousands to millions of single cells to be processed in a high throughput manner.
The ability to understand the complexity of a cell population requires analysis from all aspects. However, multiplexing of single-cell assays can be tricky, as many analysis techniques destroy the cells in the process—leading to inaccurate results.
It can be difficult to discriminate the biological noise in a heterogeneous population. Single-cell analysis can help to overcome this problem in standard assays.
As the methods and platforms for single-cell analysis advance, the data produced increases significantly. Analyzing detailed data generated from a single cell is a challenge, for example single-cell sequencing provides vast arrays of information, requiring specialist bioinformatic analysis and interpretation. For the majority of data, it is difficult to link together the key phenotypic or molecular response.
LL: What can researchers do to overcome these challenges?
MR: New technologies are being developed to isolate cells in a gentle manner to help ensure cell viability. These include cell printers and microfluidic platforms that harness picodroplet technology, such as the Cyto-Mine® Single Cell Analysis System (Sphere Fluidics), which protect the individual cells from shear stress.
Having the flexibility to perform multiple assays on a single cell, or continuous assessment of a single cell, can provide insight into its dynamic changes.
Furthermore, the exploitation of artificial intelligence in single-cell analysis may be able to detect trends in data, for example diagnostics to support the early detection of disease.
LL: Could you highlight some applications of single-cell technologies?
MR: The list of applications is extensive; many industries benefit from understanding the nuances of an individual cell or have requirements for populations derived from a single clone.
Within biopharmaceutical discovery and development, there is an inherent need for single cells in the production of biologics. Ensuring that biologics are derived from a single clonal source has been stipulated by the International Council for Harmonisation of Technical Requirements for Pharmaceuticals for Human Use (ICH) in order to ensure consistency and quality of production, reduce population heterogeneity, and minimize genomic instability. Similarly, induced pluripotent stem cells should be derived from a single progenitor to ensure the resulting population has the same genetic background.
One of the key applications where single-cell technology can be harnessed is in the detection of rare cells from large heterogenous populations. This enables the screening and isolation of valuable, rare B cells for antibody discovery, and high-expressing single cells for cell line development, helping to increase the speed and efficiency of entry into the biologics supply chain. In addition, single-cell analysis can be used in diagnostics to detect circulating tumor cells which appear in very small numbers hidden amongst millions of red blood cells and leukocytes in peripheral blood.
The use of single-cell technology is greatly advancing our understanding of the gut microbiome. Single-cell sequencing of microbial cells has the potential to identify specific functions of gut bacteria, providing insights into how the complex variation of microbial strains influences disease. The same can apply to environmental applications, such as remediation and screening for rare soil bacteria with required characteristics, and the screening of marine microbes. Finding the “right” microbes from this source could prove to be beneficial for a variety of applications.
Other areas of application include use of synthetic biology to produce valuable clones, precision genome editing and investigating cell-cell interactions to elucidate mechanisms of disease or discovery of new targets.
LL: What techniques are currently available for identifying single cells and could you touch on some of the advantages and drawbacks related to each technique?
MR: There are four techniques currently in use for the analysis of single cells, namely single-cell imagers single-cell sequencing, flow cytometry and microfluidic droplet technology platforms.
Single-cell imagers
These usually scan single cells or populations of cells to identify specific cell types or, with fluorescent or colorimetric probes, the presence of specific proteins or cell features. They are valuable for phenotype identification but are usually very slow and some can be quite expensive.
Single-cell sequencing
Single-cell sequencing delivers data-rich outcomes, which provide a detailed insight into the heterogeneity of gene expression across a population following bioinformatic analysis. The throughput is relatively low, with approximately only a few hundred cells being analyzed per experiment. However, when combined with microfluidics, this can vastly improve the throughput to tens of thousands, but this is still relatively low compared to other techniques.
Flow cytometry
Flow cytometry is a highly established technology with incredibly high throughput, measuring approximately 70,000 events per second. It offers some assay capabilities. However, it is not possible to carry out cell secretion assays with confidence as any secreted molecule can diffuse into the extracellular environment. While cold capture secretion assays do provide an insight into the secreted proteins that are retained on the cell surface, these are not measuring in real time. Another disadvantage of flow cytometry is that the procedure is reliant on labeling the cell or target of interest before processing, requiring previous knowledge of the target. In addition, the high shear forces applied during sorting may result in loss of fragile primary cells.
Microfluidic droplet technology
Microfluidic droplet technology offers high-throughput single-cell analysis on a miniaturized scale, along with assay capabilities. The droplet provides a micro-reaction chamber that traps secreted antibodies or other secreted biomolecules, allowing for ultra-sensitive and rapid assays in comparison to flow cytometry, which can only measure cell-surface associated or diffused molecules.
Droplet technology can be complex to employ into integrated easy-to-use platforms. However, the Cyto-Mine®, which uses Sphere Fluidics’ patented picodroplet technology, is an automated platform that combines single cell screening, sorting, dispensing, imaging and clone verification. It can process up to 40 million cells per day, and assesses and isolates those that produce a specific antibody or protein to help streamline workflows and improve throughput in biologics discovery and cell line development.
Dr Marian Rehak was speaking with Laura Elizabeth Lansdowne, Science Writer for Technology Networks.