pH Testing and the pH Meter for Food Analysis
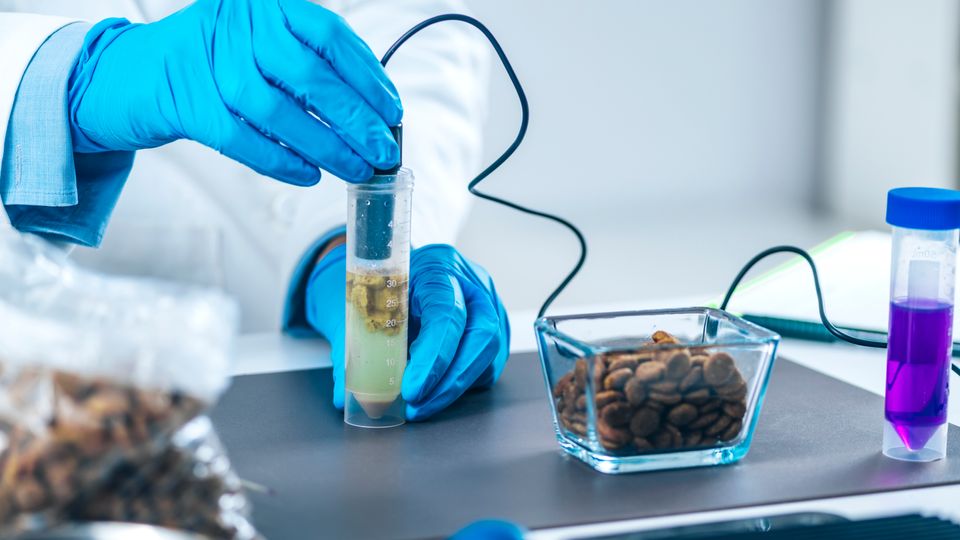
Complete the form below to unlock access to ALL audio articles.
pH is a measurable parameter between the values of 0 and 14, defining the acidity or alkalinity of a solution. Solutions with a pH = 7 are considered neutral, pH < 7 are considered acidic and pH > 7 are considered basic, or alkaline. The term pH can be defined as the “potential of the hydrogen ions” and its value determines many factors in the chemical environment of molecules, affecting properties such as the solubility of compounds, chemical reactions, availability of nutrients, biological functions and microbial activity among many others. In this article, we will consider how pH can be measured, what it tells us and its role in the food industry.
What is pH testing?
How does a pH meter work and what does it tell us?
Different types of pH and acidity testing, including titration
- pH indicators
- pH strips
- Titration
- Volatile acidity
Why do we measure the pH of food?
Applications of pH testing in the food industry
pH food chart
- What are acidic foods?
- Alkaline diet, accuracy of alkaline food list, including alkaline fruits
What is pH testing?
pH testing is the process by which the acidity or alkalinity of a substance it determined. Expressing the acidity of a solution imposes challenges as it involves a single ion species quantification, over a broad range of concentrations. Therefore, a more convenient way to express this property was established based on a logarithmic measure of the concentration of hydrogen ions in a solution.
According to the Arrhenius theory of acids and bases, substances can be classified based on what kind of ions they form when dissociated in water. I.e., an acid is any substance that, when dissolved in water, produces dissociated hydrogen (H+) ions or hydronium ions H3O+, and a base is a substance that, when dissolved in water, produces dissociated hydroxyl (OH-) ions.1 In this context, the pH scale quantifies the H+ ions in a solution, being defined by the logarithm of the reciprocal hydrogen ion activity, (αH+) according to the following equation:
pH = -log10 (αH+) = log10 (1/αH+)
A pH meter is an electric device used to measure hydrogen ion activity in water-based solutions.2 The pH meter is used in many applications such as for laboratory experimentation, in testing food and beverages, in the oil and gas industry, for testing pharmaceuticals, verifying water treatments and in agriculture among others. Figure 1 represents the pH scale and the placement of common products and substances.
Figure 1: pH scale and representation of common products according to their pH values. Credit: Technology Networks.
How does a pH meter work and what does it tell us?
The pH meter measures the difference in electrical potential (or voltage) between electrodes placed in the solution to be tested. The more acidic a solution, the more positively charged H+ ions are present and therefore the greater the electrical potential it has to produce an electrical current. This measurement can be performed through a variety of designs, but always includes two electrodes that can be individual or integrated in a single probe. The most modern and compact designs consist of a single glass probe (see Figure 2) containing the two electrodes, an internal reference electrode and external sample electrode. These electrodes are usually made out of silver wire covered with silver chloride, and the probe is filled with a buffer solution of potassium chloride (KCl) at pH 7. The measured pH is the difference in H+ activity between the reference buffer inside the probe and the sample solution.2
Figure 2: Representation of a pH probe. Credit: The Author.
Because pH measurements are performed with respect to a reference, calibration is crucial to attain an accurate measurement. Calibration ensures that the voltage output of the pH electrode correctly correlates with pH values of known solutions. Furthermore, the pH calculation is dependent on the temperature.3 Temperature compensation is then critical for accurate results. This compensation is performed by a temperature sensor in contact with the test solution or in some designs, integrated in the measuring probe.
Measuring protocols and probe geometry can be adjusted according to the measuring method.4 The simplest form of measurements are in clear liquid samples or with low suspended solids, such as clear juices, wine or vinegar. In these samples, direct measurements are performed by inserting the probe into the liquid and reading the meter. Direct measurements can also be performed in gels or semisolid food products such as in cheese, yogurt or jams. These determinations are usually performed with a conical probe (or insertion probe), introduced directly into the product. Direct measurements in gels or semisolids usually takes longer to achieve a stable reading and are only valid for samples that are uniform. In samples where these measurements are not possible, the testing sample can be diluted or blended in distilled water according to specific protocols. After dilution and blending, the solids are removed by filtration or centrifugation and the liquid fraction can be tested. Additional probe designs are available, such as the “extra thin” probe for trace amounts of sample or the “flow-through” type that allows continuous inline measurements.
Overall, the pH meter is a versatile piece of equipment, easy to control and operate and produces rapid and reproducible measurements. There are portable pH meters, so they can be easily transported and used in-field. However, special care must be taken with the probe´s cleaning to avoid sample contamination and to avoid damage to the fragile probe structure.
Different types of pH and acidity testing, including titration
The pH meter is the most versatile and reliable method to determine pH, being thus considered the gold standard for pH testing.3 However, there are other methods available to determine pH and acidity that may be more suitable in certain processes or that require less advanced equipment and involve lower costs.
pH indicators
Acid–base indicators, or pH indicators, are substances that change color according to the solution´s pH. These compounds are usually weak acids or bases that, when dissolved in water, dissociate slightly and form ions without significantly influencing the solution´s pH.2 Some indicators change color, while others change from colored to colorless states. Examples of common pH indicators are phenolphthalein (colorless < 8.3, between pH values of 8.3 and 10 it is purple-pink, turning colorless again from 12 on) or methyl red (red < 4.4, between 4.4 and 6.2 it is orange and yellow > 6.2). There are also naturally occurring pH indicators, such as anthocyanins found in flowers, fruits and vegetables or Litmus dyes, which are a mixture of pH sensitive dyes extracted from lichens. These natural pH indicators are currently used as a natural, food grade alternative to synthetic dyes in applications such as pH-sensitive smart food packaging.5
Different indicators can be combined to give a gradual change in color over a wide pH range, creating a universal indicator. The pH of a solution can then be approximately identified when a universal indicator is mixed with the solution. Alternatively, precise measurements can be performed by using absorbance spectroscopy to calculate the pH indicator using Beer–Lambert law,6 that correlates the light absorption of a material with properties such as the concentration of a compound. Spectroscopic pH determinations using a single acid–base indicator are highly accurate, while combining two or more indicators causes the accuracy to be decreased.
pH strips
pH test strips are based on the Litmus method, involving a mixture of pH sensitive dyes fixated in paper strips. The substances in the paper show different colors at different acidities that can be compared with a reference chart (Figure 3). Some pH test strips can measure a pH from 0 to 14, but there are also pH test strips that can measure only acid or only alkaline substances but do so more precisely. Test strips are easy to use, inexpensive, disposable and provide fast results. However, test strips provide low accuracy and experimental errors are common, particularly as the technique relies on the color perception of the operator.7 Because of this, pH test strips are a great solution for fast and low budget determinations, where accuracy is not paramount and no safety constrains are involved. For any other applications, a more precise and reliable method must be used.
Figure 3: Example of a pH strip reference chart. Credit: The Author.
Titration
In the context of food analyses, there are two interrelated concepts that deal with acidity: pH and titratable acidity. We have already established that pH measures the concentration of dissociated H+ ions, while the titratable acidity measures the sum of free protons and un-dissociated acids in a solution. In other words, the total titrable protons in a substance or its ability to neutralize a base.8 This parameter is important since it correlates better with the sensorial properties of food than the pH value.
Titrable acidity is determined by adding a standard basic solution to a known amount of test sample. The endpoint of the titration is determined either by a target pH value measured by a pH meter or by the color change of an indicator dye (see Figure 4), most commonly phenolphthalein. By considering the added volume of the standard base and its concentration, the concentration of the titrable acids on the sample can be found, being expressed as the concentration (g/L) of the predominate organic acid (e.g., lactic acid in dairy products). There are several standard methods to determine the titrable acidity in different foods; however, the sample requirements and preparation are similar to the ones described for the pH meter measurements.4 The sample amount must be known, suspended solids must be avoided and in the case of using a dye, particular care must be taken with the sample’s color as it can hinder the color perception at the indicator turning point. The general layout for titrable acidity testing involves a beaker to hold the sample, a magnetic stirrer to ensure homogeneity and a burette to gradually add the standard base.
Figure 4: Representation of a titration layout using a pH meter to determine the endpoint (A) or using a pH indicator to determine the endpoint by the color change (B). Credit: The Author.
Volatile acidity
Volatile acidity is a measure of volatile acids in food products. It is most commonly performed on wines and other alcoholic drinks as an indicator of the product´s quality and spoilage. The values are expressed as the concentration of acetic acid, since it is the largest contributor to this parameter, but other acids such as lactic, formic, butyric and propionic acids can contribute.9 Volatile acidity relates more with acid detected by their aroma than by the palate, so the quality of many food products is related to the content of volatile fatty acids. Therefore, the volatile acidity is an important parameter in, e.g., assessing butter quality and following cheese ripening. Commonly, the volatile acidity is determined by distilling the volatile acids and determining their concentration through titration. Nowadays, more advanced techniques (i.e., gas chromatography), are available, allowing more precise determinations and the identification of the different volatile acids present in the samples.
Why do we measure the pH of food?
pH is highly influential in food and beverage production and its variability can cause critical disparities in quality, consistency and safety of the food products. For these reasons, pH is one of the parameters most frequently controlled during production and quality control before release.
The pH values largely affect bioprocesses driven by enzymatic or microbial activity, impacting process yields and specificity. Taste is radically influenced by pH, since pH levels can help to regulate sweetness and sourness perception. In addition, the texture is pH dependent and many foods (e.g., cheese, jams and creams) rely on accurate pH levels to create and control the desired texture. pH level also affects food appearance, by stabilizing or destabilizing colloidal mixtures and thus leading to the formation of a hazy appearance or precipitates in clear mixtures. Many food constituents are also natural pH indicators, changing color according to the pH. This is the case of anthocyanins in wine, fruits and vegetables.10 In food safety, pH plays a crucial role in inhibiting the growth of spoilage microorganisms. For these reasons, most regulatory agencies impose rules for the pH levels of certain commercial foods, since generally acidic foods (pH < 4.6) are considered safer and allow longer shelf lives.11
Applications of pH testing in the food industry
pH testing reaches all the areas and stages of food production, being one of the most common and important analytic methodologies in the food industry. Almost every aspect in food manufacturing can be modified by small changes to pH levels, with implications for food safety and quality.
- Food safety
Perhaps the main concern of the food industry is food safety. In this context, pH control is vital in ensuring the physicochemical and microbiological safety of foods. Most industrial facilities rely on standardized procedures to ensure adequate hygiene. These procedures often include the use of strong acids and bases to clean deposits, pipelines and food processing equipment through several cycles of washing and rinsing. pH testing, either by pH meter or by using indicators, is a standard procedure to ensure proper removal of the dangerous cleaning products from the production lines. Furthermore, pH levels regulate the type of microorganisms that can grow in food products; the more acidic the food product is, the more difficult it is for microorganisms to survive and grow in it. - Quality control
Naturally, pH testing is essential to guarantee that food products fall into the required specifications. This is valid for the production of fruits and vegetables, where pH and acidity are fundamental factors to access the quality of the product.12 Also in the control of raw ingredients, final products and even during storage, pH testing will ensure that foods meet the required specifications. - Process control and optimization
As previously addressed, pH control is fundamental to enzymatically and microbially driven processes. Wine fermentation and aging are extensively influenced by the pH since, at low pH values the yeast activity would be compromised, and at higher pH values the must and wine would be susceptible to spoilage bacteria. The beer brewing process is also dependent on the accurate control of pH, not only in the fermentation, but also during the entire process. In the mashing stage, enzymatic activity is regulated by the pH and affects the degradation of starch into fermentable sugars, and thus the overall process yield. Adequate pH levels also result in better hop extraction and clarity, with a less hazy appearance of the final product.
The dairy industry also offers a common example of the importance of pH testing. During cheese and yogurt production, the coagulation of the milk proteins is influenced by the pH, affecting both the process yield and final texture. Likewise, both production processes involve microbial activity that strongly influences the product’s flavor, aroma and shelf life. From the technological point of view, pH monitoring can be used as a process control parameter. Taking the previous examples, making yogurt mostly consists of lowering the pH value of milk to induce protein coagulation. By monitoring the pH, it is possible to know when the process is completed without sampling the product or disturbing the product process. - Research and development
Ultimately, pH reflects the chemical conditions of a solution. This parameter can control the availability of nutrients, biological functions of macromolecules, microbial activity and the behavior of chemicals. Because of this, monitoring or controlling the pH is essential in food science and technology and it is carefully performed in researching, developing and testing food ingredients, products and processes. An excellent example of this is the importance of pH control when dealing with proteins.13 Proteins are the structural and functional part of many food products. They are dynamic macromolecules, highly responsive to the environmental conditions and pH is the most fundamental factor affecting their behavior and performance. The surface charge of a protein (and often its structure) is pH dependent, thus its solubility, emulsification, gelation, thickening, foaming and water-binding capacity and ability to interact with other molecules are regulated by the pH.14 An accurate control of the pH critically defines the success and performance of any food formulation dependent on these and other properties.
pH food chart
What are acidic foods?
Foods may have low pH because of naturally occurring acids, and as a result, most foods are considered acidic (Table 1). Through scientific criteria, any food with a pH lower than 7 is considered acidic, however there are subclassifications according to the acidity level. Food products with a pH between 4.6 and 7 are considered low acid foods and are more susceptible to microbial growth (e.g., fungi, yeasts and pathogenic bacteria, such as Clostridium botulinum).15 For this reason, common production standards and regulatory legislation often requires the use of severe preservation processes in such products. High acid foods, such as most fruits and fermented foods, have a pH naturally below 4.6, ensuring higher safety and demanding lower protection concerns. Similarly, safety criteria are applied to acidified foods, achieving a pH value below 4.6 by the addition of edible acids.16 To ensure adequate pH levels, increase shelf life and prevent spoilage, manufactures often include ingredients to regulate acidity, such citric or acetic acid either in their pure forms or as lemon juice and vinegar.
Table 1: Example of pH values of some common foods.
Food | pH |
Apple | 3.30 - 4.00 |
Banana | 4.50 - 5.20 |
Beans | 5.70 - 6.20 |
Beef | 5.30 - 6-00 |
Bread, white | 5.00 - 6.20 |
Cabbage | 5.20 - 6.80 |
Carrots | 5.88 - 6.20 |
Cheese | 4.75 - 7.50 |
Chicken | 5.80 - 6.40 |
Coffee | 4.85 – 5.10 |
Eggs | 6.58 - 8.00 |
Fish | 6.50 - 6.80 |
Lemon | 2.00 - 2.60 |
Oranges | 3.10 - 4.10 |
Potato | 5.40 - 5.90 |
Soy milk | 7 |
Tea | 7.2 |
Tomatoes | 4.30 - 4.90 |
Alkaline diet, accuracy of alkaline food list, including alkaline fruits
An alkaline diet has been popularized, claiming to improve health, fight disease and reduce cancer risk. The basic claim is that such a diet improves the acid–base balance and raises the urinary pH. However, these claims are not backed by science and are based on unsound premises. Some advocates of this diet argue that the classification is not related with the food´s actual pH but their effects on the body or on the abundance of alkali-generating ash or mineral content in such foods. This does not change the fact that the classification of acidic and alkaline foods promoted by this current trend is not really related with their actual pH values. Thus, the common tables and charts categorizing foods according to the alkaline diet criteria are fundamentally erroneous. An example of one such "alkaline food" chart can be seen in Figure 5.
Figure 5: Representation of food products according to the “alkaline diet” concept. Credit: Technology Networks.
In fact, as can be perceived by the data in Table 1, the vast majority of foods are acidic or neutral and the information provided in examples such as Figure 5 do not reflect the actual pH of the foods. The distribution of the food in these charts appears more related with a qualitative perception of their impact in health, e.g., fruits and vegetables are placed on the alkaline side, while food with high fat and sugar content, such as fast food and candy, are placed on the acidic part. Few food products display an alkaline pH, among which eggs, some shellfish and baked products such as pretzels are some examples. Pretzels in particular display the highest pH values, since during their manufacturing, these products are immersed in baking soda or sodium hydroxide solutions. Furthermore, the claim that the alkaline diet can change the body´s pH is dubious, as there are no studies proving it to be so and the human body has several mechanisms to maintain a stable pH level. Yet, and regardless of the scientific inaccuracies and little dependence on the food pH, the “alkaline diet” does encourage healthy eating patterns, focusing in fresh fruits, vegetables and plant-based alternatives.17 The limitation of animal protein intake, associated with the guidelines of the alkaline diet may also lead to the reduction of acidic compound accumulation in the body and possibly improve renal function.18 Regardless of the diet’s effectivity or eventual health benefits, its classification as well as the pH values of foods included on its lists should be approached with caution and with awareness that it does not follow the scientific criteria for pH determinations and classification.
References
1. Sadler GD, Murphy PA. pH and titratable acidity. In: Nielsen SS, ed, Food Analysis. 4th Ed., Springer; 2010:219-238. doi:10.1007/978-1-4419-1478-1_13
2. Karastogianni S, Girousi S, Sotiropoulos S. pH: Principles and measurement. In: Caballero B, Finglas PM, Toldrá F eds. Encyclopedia of Food and Health. Vol 4. Elsevier; 2016:333-338. doi:10.1016/B978-0-12-384947-2.00538-9
3. Buck RP, Rondinini S, Covington AK, et al. Measurement of pH. Definition, standards, and procedures (IUPAC Recommendations 2002). Pure Appl Chem. 2002;74(11):2169-2200.
4. FDA. Title 21 - Food and drugs chapter I - Food and drug administration department of health and human services subchapter b - Food for human consumption. U.S. Department of Health and Human Services. https://www.accessdata.fda.gov/scripts/cdrh/cfdocs/cfcfr/CFRSearch.cfm?fr=114.90. Published November 29, 2022. Accessed January 19, 2023.
5. Alizadeh-Sani M, Mohammadian E, Rhim J-W, Jafari SM. pH-sensitive (halochromic) smart packaging films based on natural food colorants for the monitoring of food quality and safety. Trends Food Sci Technol. 2020;105:93-144. doi:10.1016/j.tifs.2020.08.014
6. Swinehart DF. The Beer-Lambert law. J Chem Educ. 1962;39(7):333-335. doi:10.1021/ed039p333
7. Metheny NA, Gunn EM, Rubbelke CS, Quillen TF, Ezekiel UR, Meert KL. Effect of pH test-strip characteristics on accuracy of readings. Crit Care Nurse. 2017;37(3):50-58. doi:10.4037/ccn2017199
8. Jennings PA, Mullen CA, Roy M. Titration and pH measurement. In: eLS. Wiley; 2010. doi:10.1002/9780470015902.a0002700.pub2
9. Considine JA, Frankish E. Essential analyses. In: A Complete Guide to Quality in Small-Scale Wine Making. Elsevier; 2014:137-154. doi:10.1016/B978-0-12-408081-2.00010-X
10. Andrés-Bello A, Barreto-Palacios V, García-Segovia P, Mir-Bel J, Martínez-Monzó J. Effect of pH on color and texture of food products. Food Eng Rev. 2013;5(3):158-170. doi:10.1007/s12393-013-9067-2
11. Siddiqui MW, Rahman MS, eds. Minimally Processed Foods. Springer International Publishing; 2015. doi:10.1007/978-3-319-10677-9
12. Anthon GE, LeStrange M, Barrett DM. Changes in pH, acids, sugars and other quality parameters during extended vine holding of ripe processing tomatoes. J Sci Food Agric. 2011;91(7):1175-1181. doi:10.1002/jsfa.4312
13. Boulet M, Britten M, Lamarche F. Aggregation of some food proteins in aqueous dispersions: effects of concentration, pH and ionic strength. Food Hydrocoll. 2000;14(2):135-144. doi:10.1016/S0268-005X(99)00059-4
14. Turgeon SL, Gauthier SF, Paquin P. Emulsifying property of whey peptide fractions as a function of pH and ionic strength. J Food Sci. 1992;57(3):601-604. doi:10.1111/j.1365-2621.1992.tb08052.x
15. Rahman MS ed. Handbook of Food Preservation. CRC Press; 2020. doi:10.1201/9780429091483
16. Clark JP. The basics about acid levels in food. Food Technol. 2014;68(7):70-72.
17. Schwalfenberg GK. The alkaline diet: Is there evidence that an alkaline pH diet benefits health? J Environ Public Health. 2012;2012:1-7. doi:10.1155/2012/727630
18. Passey C. Reducing the dietary acid load: How a more alkaline diet benefits patients with chronic kidney disease. J Ren Nutr. 2017;27(3):151-160. doi:10.1053/j.jrn.2016.11.006