Overcoming Western Blot Reproducibility Problems: Tips for Producing More Accurate and Reliable Data
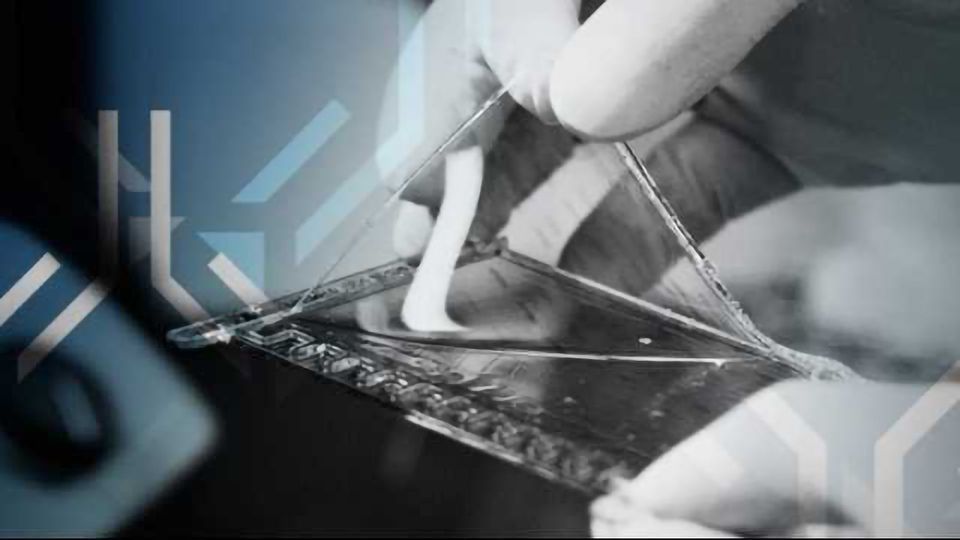
Complete the form below to unlock access to ALL audio articles.
The goal of western blotting is to reliably detect and quantify protein expression levels. When performed well, they can be accurate and cost-effective.
But, according to a Bio-Rad survey of North American scientists, nearly half reported that their western blots fail at least a quarter of the time. Furthermore, seven out of ten who measured variation reported that their results weren’t reproducible (%CV > 10%). These results suggest that a majority of scientists find it challenging to accurately quantify their western blots and generate reliable data.
This article outlines key workflow steps to help researchers perform better western blots. By executing best practices in these steps – sample preparation, gel electrophoresis, gel transfer, protein detection, and image analysis – researchers can expect good western blot data.
STEP ONE: Optimizing Lysis During Sample Preparation is Critical
During sample preparation, scientists should compare lysis methods—the methods used for breaking open the cells—and optimize the lysis buffer to maximize solubility and recovery of the target protein(s). Moreover, contamination and protein degradation need to be minimized.
Clean tools, and pH-neutral water will keep contamination down, and cold materials will slow protein degradation. That means working with ice-cold buffers and processing tissues immediately or flash-freezing and storing tissues at -80 °C.1
Scientists can optimize their lysis buffers by considering the cellular location of the protein.1 For example, RIPA lysis buffer is preferred for extracting nuclear proteins. If the target protein yield is low, scientists should consider fractionation to enrich for low-abundance proteins.1
A wide range of cell disruption methods exists. Detergent-based or enzymatic-based methods are gentle and suitable for extracting protein from cells. Harsher methods such as sonication or French-pressing are normally required to disrupt tissues. With harsher methods, foaming should be avoided because it can decrease protein yield.1
Lysis releases proteases that can affect protein stability. A cocktail of protease inhibitors is added to prevent protein degradation. For best results, the lysis buffer recipe should be adjusted according to the requirements of the target protein(s). For example, to preserve SUMOylation, it’s best to add isopeptidase inhibitor proteins, and for ubiquitylation, ethylenediamine tetraacetic acid (EDTA) or ethylene glycol tetraacetic acid (EGTA) and iodoacetamide (IAA) or N-ethylmaleimide (NEM) should be added to the lysis buffer.1
STEP TWO: Gel Electrophoresis with the Right Materials
Electrophoresis separates the proteins contained in the cell extract by size. For optimal separation, scientists should consider the gel type, the chemistry of the gel and the running buffer, and the total amount of protein in their sample.
The gel type is selected based on the molecular size of the target protein(s).2 Gels with a higher percentage of polyacrylamide (10% or more) have smaller pores and can separate small proteins (<15 kDa). Proteins with higher molecular weights should pass through gels with lower polyacrylamide percentages. Gradient gels (in which polyacrylamide percentage increases from top to bottom) can separate proteins with a wide range of molecular sizes.
Many types of buffers are used for gel electrophoresis. For example, Tris-acetate gels and XT or Tricine-based buffers offer the best separation for large proteins (>250 kDa), for peptide sequencing, or mass spectrometry applications. Tris-HCL gels with Tris/glycine/sodium dodecyl sulfate (SDS) buffers are cheap and easy to prepare in-house. If a long shelf-life is desirable, TGX gels have a one-year shelf life.3
To avoid under- or over-loading a gel and to ensure accurate quantification for downstream steps, scientists should measure the total protein in their samples before loading the gel. Commercial kits are available to measure the total protein concentration in samples. To compare differences in protein levels, it is best practice to load samples within the linear range of detection.4 To do this, a dilution series test is run to determine the range of sample loading amounts that provide a linearly proportional protein signal on the gel.
STEP THREE: Transfer Depends on the Blotting Membrane and Transfer Method
In this step, proteins are transferred from the gel and onto a membrane using electrophoresis. There are two important aspects: selecting the type of blotting membrane and transfer methods.
Polyvinylidene difluoride (PVDF) or nitrocellulose membranes are used in western blotting. PVDF membranes have higher mechanical strength for stripping and re-probing, can transfer hydrophobic or membrane proteins, and work well with SDS-containing buffers, but require pre-soaking in methanol. Nitrocellulose membranes have excellent signal-to-noise ratios and do not require methanol pretreatment. However, they are brittle and SDS-containing buffers inhibit protein binding.3
There are a few options for transfer methods:3
- Wet: The most popular method, wet transfers are flexible in terms of voltage, blotting times, cooling, and protein sizes.
- Semi-Dry: With transfer times of 15–60 minutes and an easier assembly, this method is preferred for its high throughput and when discontinuous buffer systems are needed. However, extended transfer times are not possible due to buffer depletion. It works best with proteins 30–120 kDa in size.
- Rapid Semi-dry: These systems complete the transfer of a broad range of protein sizes in as little as three minutes. They achieve this through the use of proprietary buffers and materials.5
For best results, researchers should optimize the transfer method and minimize handling of the membrane.
STEP FOUR: Maximizing Signal with Good Antibody Practices
For western blot success, a good primary antibody must be sensitive (offer a strong signal to the target protein) and specific (does not bind to other proteins). The best commercial antibodies provide performance data on a variety of cell lysates and recommend concentrations for use, but scientists should empirically determine the concentration for best results.6 To confirm that the primary antibody is specific, scientists must include suitable positive and negative controls. A positive control may include a cell or tissue lysate that expresses the target protein(s). A secondary antibody (no primary antibody) sample and a sample from a cell or tissue lysate which is known not to express the target protein(s) can work as negative controls.
Different agents can be used for blocking non-specific sites including homemade recipes as well as commercially available preparations. Since each antibody-antigen interaction is unique, care must be taken to ensure that the blocking agent is compatible. For example, non-fat milk should not be used in the detection of phosphoproteins, biotin-avidin/streptavidin or alkaline phosphatase. Another example, BSA is not recommended for use with antibodies created using BSA-coupled peptides or with phosphotyrosine antibodies.3
To detect multiple proteins on the same blot, common practice is to strip and reprobe or to cut the membrane into individual strips for detection with different antibodies. But with fluorescent detection, multiplexing for multiple targets simultaneously is possible and obviates the need for stripping or cutting the membrane, thereby saving time and conserving the sample.7 Additionally, multiplex detection makes it possible to detect both phosphorylated and non-phosphorylated forms of a protein at the same time, something not possible when cutting membrane into strips. Fluorescence western blotting also offers a linear range of detection of up to six orders in magnitude. Newer fluorescent labels composed of polymer nanoparticle fluorophores provide a brighter signal than conventional fluorescently labeled antibodies and offer comparable sensitivity to chemiluminescence detection.8
STEP FIVE: Image Analysis and Normalization Complete the Western Blot Workflow
In this step, the size, quantity and purity of proteins is measured.
Many kinds of software can be used for image acquisition and quantification of protein bands from western blots. During image analysis, first, scientists designate the regions on the blot that will be quantified and subtract background.9 There are two main methods for background subtraction. In general, the ‘lanes and bands’ method provides a finer control compared to measuring volume boxes. After background subtraction, scientists need to normalize western blot data to enable a robust comparison between the levels of protein expression in the samples.
Normalization accounts for inconsistencies during sample preparation, gel loading, and gel transfer, ensuring that the protein levels detected are the direct result of the experimental conditions.10 Traditional house-keeping protein (HKP) normalization is a solid approach if it can be verified that HKPs do not vary with experimental conditions, and they are not used at saturating levels. Total protein normalization (TPN), which uses protein in the entire lane to normalize expression levels, is an emerging technique that is both simpler and faster.11 There is also a new stain-free TPN method that saves more time and is more sensitive than the traditional Coomassie Blue staining method.12
Conclusion
Western blotting is often a multi-day workflow that requires making several decisions and executing several laboratory steps properly. To collect good western blot data, the focus should be on optimizing each of the steps described to the target protein(s). From there, scientists should consider alternative steps to simplify or to automate the workflow without sacrificing reproducibility, while keeping the overall method consistent to minimize variability and increase confidence in the results.
References
1. 10 tips for western blot detection of proteins present in tissue lysates, Bioradiations, accessed October 17, 2018
2. Tips for selecting the best gel for your application, Bio-Rad Laboratories, accessed October 17, 2018
3. Best practices for the best western blots, Bioradiations, accessed October 17, 2018
4. Western blot normalization methods, Bioradiations, accessed October 24, 2018
5. Lin-Moshier Y and Marchant JS. A rapid western blotting protocol for the Xenopus oocyte, Cold Spring Harb Protoc. 2013 (3), doi: 10.1101/pdb.prot072793
6. The ABCs of finding a good antibody: How to find a good antibody, validate it, and publish meaningful data, F1000 Research, accessed October 17, 2018
7. Kondo Y, Higa S, Iwasaki T, Matsumoto T, Maehara K, Harada A, Baba Y, Fujita M, Ohkawa Y. Sensitive detection of fluorescence in western blotting by merging images, PLoS One. 2018 Jan 19;13(1):e0191532. doi: 10.1371/journal.pone.0191532.
8. Fluorescent western blotting antibodies, Bio-Rad Laboratories, accessed October 17, 2018
9. Turner L, Oh K. The how and why of normalizing your western blots, Bioradiations, , accessed October 17, 2018
10. The new normalization in western blotting, Technology Networks, accessed October 17, 2018
11. ImageLabTM Total Protein Normalization Basic Tutorial, Youtube, accessed October 17, 2018
12. Yadav G, Oh K. Defining the New Normal in Quantitative Western Blot Data, Bioradiations, accessed October 17, 2018