Neurotechnology
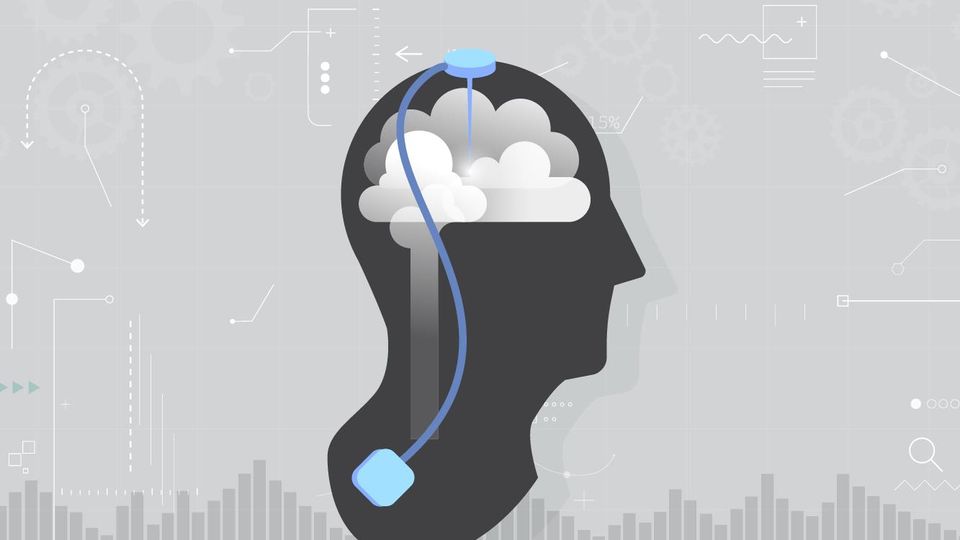
Complete the form below to unlock access to ALL audio articles.
Imagine you could control your computer's mouse with your brain instead of your hand1. Imagine helping a patient with spinal cord injury to walk again2 by using a brain implant.
It might sound like science fiction, but researchers have figured out how to make both scenarios a reality. It's all thanks to neurotechnology.
There are many different types of neurotechnology, each with their role to play. From improving therapeutics for neurological and psychiatric disorders to augmenting current human capability (ever wanted to read someone's mind?) neurotechnology has a wide range of applications that make it a blossoming field worth paying attention to.
What is neurotechnology?
In its simplest form, neurotechnology is the integration of technical components3 with the nervous system. These components can be computers, electrodes or any other piece of engineering that can be set up to interface with the electric pulses coursing through our bodies.
Neurotechnology has two main objectives - either to record signals from the brain and “translate” them into technical control commands (like our brain-controlled computer mouse), or to manipulate brain activity by applying electrical or optical stimuli (to help our paralysis patient).
The applications of neurotechnology are wide-ranging - from furthering the potential of academic research, to therapeutics, to developing brain/machine interfaces and more - and there are a lot of different types of neurotechnologies, some less invasive than others, which we will cover in this article.
Electrophysiology
In the 1780s, while experimenting with frogs’ legs, Luigi Galvani noticed that applying electric shocks made the legs twitch4 - even though they were disconnected from the frog's brain and body.
That breakthrough instigated two centuries of research which would teach us a substantial amount5 about how neurons fire in response to stimuli, and how that firing is carried across different areas of the brain. It was the key that unlocked our understanding of how the brain is organized6.
In a nutshell7, electrophysiology involves the use of electrodes to understand the electrical properties of neurons. Researchers can record the activity of hundreds of cells at once, or home in on single cells using the patch-clamp technique.
An electroencephalogram (EEG) [1] is a type of electrophysiological monitoring method used to record electrical activity of several neurons at once. It is typically noninvasive, with the electrodes arranged in a cap and placed over the scalp, which measures voltage fluctuations of the brain regions beneath8. By contrast, an electrocorticogram (ECoG) involves placing electrodes in direct contact with the surface of the brain and measuring the brain waves in those specific brain regions. It is typically used intraoperatively to map epileptic regions of the brain and facilitate their safe removal9.
Figure 1: Patch-clamp electrophysiology
In patch-clamp electrophysiology10, a glass micropipette with diameter < 3 microns11 is inserted into the membrane of a single cell. Electrically charged ions passing from the inside to the outside of the cell through the trans-membrane channels charge the pipette solution. The electric current generated by this transmembrane movement of ions is detected by a metal electrode, which relays the data to an amplifier. This technique gives researchers incredible precision and certainty in their readings.
Researchers can also measure the activity of several neurons at once. There are two main ways of doing this12. Firstly, a microelectrode array can be used. This is a grid of dozens of electrodes which can record the activity of multiple neurons on the surface of the brain. Despite being small in size, it is still too large to be inserted deep in the brain, so this technique is reserved for neurons on the surface of the brain.
The second technique involves tetrodes. Tetrodes are microelectrode arrays composed of just four active electrodes - making them small enough to insert into these deeper regions.
Measuring large swathes of neurons13 like this leads to more uncertainty. Some types of neurons have a distinctive waveform, making them easily identifiable. However, these are the exception rather than the rule. Most neurons have ambiguous waveforms, making it difficult to ascertain exactly which neurons have been studied.
Deep brain stimulation
Deep brain stimulation refers to a technique14 that involves surgically implanting an electrode into specific areas of the brain to modulate the way it operates. These electrodes produce electrical impulses that regulate abnormal neuronal activity in the patient.
The stimulation delivered to the brain is regulated by a pacemaker-like device that is implanted under the skin in the upper chest. A wire runs under the skin from this pacemaker to the electrodes. Though highly invasive, this procedure is reversible15 and generally doesn't lead to many side effects.
Figure 2: A deep brain stimulator includes a pacemaker connected to electrodes in the brain.
While the exact mechanism of action isn't clear16, the therapeutic effects17 of deep brain stimulation can be significant.
For example, implanting electrodes into the ventral intermediate nucleus of the thalamus has been shown18 to dramatically decrease tremor, and even halt disease progression in essential tremor patients for more than 6 years after implantation.
Additionally, stimulation of either the internal segment of the globus pallidus or the subthalamic nucleus has been shown19 to decrease the symptoms of bradykinesia, rigidity and gait impairment in patients with Parkinson's Disease. Other conditions that benefit from treatment with deep brain stimulation include epilepsy, OCD and dystonia.
Transcranial magnetic stimulation
Transcranial magnetic stimulation (TMS)20 is a recently developed technique used in the treatment of psychiatric and neurological disorders. It belongs to a growing field of non-invasive brain stimulation (NIBS)21 techniques.
TMS exposes the scalp to a magnetic field, which can modulate the electrical signals fired from neurons in the target region. Usually the magnetic field emanates from a "wand-like" device.
Though the exact biological mechanism of TMS is not understood, it has been shown to provide relief22 from depressive symptoms and improve mood in some patients.
Transcranial direct current stimulation
Transcranial direct current stimulation (tDCS) is a method of brain stimulation23 that centers on modulating behavioral and cognitive processes, as well as the neural circuits underlying motor function.
Like TMS, tDCS is a painless and non-invasive procedure. Two electrodes are placed on the scalp of the participant - a smaller target electrode on one hemisphere, and a larger reference electrode on the other hemisphere. A weak electrical current passes from the target electrode, through the brain, to the reference electrode - and in doing so, modulates the behavior of the patient.
One line of study currently in the spotlight is ADHD therapy. Cognitive control tasks rely on good prefrontal cortex function - the impairment of this region can lead to impulse control issues. Studies have found that adolescents with ADHD exhibit reduced activity in certain prefrontal cortex regions, specifically the left dorsolateral prefrontal cortex (DLPFC). Using tDCS to stimulate the left DLPFC has been shown to reduce impulsivity in patients with ADHD, by effectively making up for the deficit in activity24.
Figure 3: tDCS may be delivered through cap-mounted electrodes.
It is generally accepted25 that a positive anodal, or excitatory, current is associated with upregulation of behaviors regulated by the brain region under the target electrode.
On the other hand, negative cathodal, or inhibitory, current is associated with downregulation of said behaviors.
tDCS is used to identify26 brain-behavior relationships across cognitive, motor, social and affective domains. Applications of tDCS on healthy populations have been demonstrated27 to temporarily modify behavior, accelerate learning, and boost task performance.
Focused ultrasound
Breakthrough research28 at Carnegie Mellon University has recently shown that low-intensity ultrasound techniques can be applied to manipulate neurons in a cell-type selective manner. In other words, focused ultrasound (FUS) gives researchers the power to modulate specific neuro-circuits, making FUS a more highly targeted neurotherapy29 than deep brain stimulation, TMS, and tDCS.
Figure 4: A diagram showing the mechanisms behind focused ultrasound (FUS).
FUS neuromodulation works by directing ultrasonic wave energy, through the skull, at highly-targeted brain regions. By tuning the parameters, scientists can either excite or inhibit specific neural circuits.
FUS is FDA-approved in the US for treatment of essential tremor30. However, it is still not widely used in hospitals - it is a relatively novel therapy, barely a decade old. It carries several advantages over older therapies for essential tremor, namely being non-invasive, not relying on radiation, and not posing any risk of infection31. For these reasons, we may see its presence pick up in the coming years.
Brain computer interfaces
Simply put, a brain-computer interface (BCI)32 is a computer-based system that receives brain signals, analyzes them and then translates them into commands for devices, which produce a desired output.
The main function of BCI in a medical context is therapeutic - restoring normal neuromuscular function to patients with disorders such as amyotrophic lateral sclerosis (ALS), cerebral palsy, stroke or spinal cord injury.
Turning brain signals into commands for a computer system means patients will be able to move a cursor, type on a keyboard, manipulate a prosthetic - just by using their brain. In 2015, researchers at the University of Houston succeeded in making an amputee control his prosthetic hand using only his mind for the first time - without the need for an invasive brain implant.
Instead, the subject wore an 64-channel EEG headset, which monitored brain activity across motor, decision-making and action observation regions of the brain. The neuronal activity in these regions preceded the movement of the prosthetic hand by 50 - 90 milliseconds, proving that the brain was anticipating the movement before it happened33.
Beyond this, BCIs also have a role to play in making surgery safer34. For example, BCIs can be used to monitor the surgeon's mental focus while they are performing a procedure, and then use this information to make the procedure safer. This system can train the surgeon to regulate their own mental state while performing surgery-like tasks using a robotic system. The system presents augmented reality feedback to the surgeon, which helps their effort in maintaining a high level of mental focus during the task.
Brain Implants
The below video shows a monkey playing Pong with its mind35. As well as being, let’s be honest, pretty strange to watch, it is a beautiful illustration of a brain-computer interface in action. This technology, developed by Elon Musk's company Neuralink, is part of a revival of interest in brain implants.
Though they might sound like something belonging to the future, the human fascination with brain implants has been around since the early 20th century, with the development of electroencephalography (EEG) by Hans Berger in 1929.36
Brain implants are one of the ways brains and computers interface in the first place. They allow users to communicate to computers and other external devices such as robotic hands. This makes them strong candidates as a therapy for patients who may have nerve damage in their limbs or spinal cord, as the brain implant allows these nerves to be bypassed entirely while still achieving the desired output.
The implants record action potentials and local field potentials of neurons with high temporal and spatial resolution and high channel count37. This lets researchers cover lots of neural tissue at once.
The implantation is currently delivered manually, by a surgeon, via a craniotomy. While it is certainly invasive, the procedure is reversible38 without any serious side-effects, at least in the pigs that Musk has also experimented on.
While Musk's vision for Neuralink is to offer its brain technology as an elective procedure39 for any average person, other brain implant companies are thinking differently.
For example, Florian Solzbacher is clear that the development of non-implantable BCIs40 are also of great interest to his company Blackrock Neurotech. According to the company, just 34 people in the world currently have a device implanted in their brains - this neurotechnology is clearly still in its infancy.
That said, Blackrock's MoveAgain BCI implant recently gained Breakthrough Device designation from the FDA, and the company intends to commercialize MoveAgain this year41. It wouldn't be a stretch to imagine we may be standing on the precipice of brain implants taking off as a standard therapy for several debilitating, chronic conditions.
Ethics of neurotechnology
If reading about all these developments in neurotechnology has made you uncomfortable, you're not alone.
Neurotechnology, while therapeutically very promising, is an ethical minefield. It raises questions around rights to data, privacy, and the risk of side-stepping regulations in the name of easier marketing.
Let's think back to our Pong-playing monkey. The researchers monitoring Pager's neuroactivity have that data on their computers. Who owns that data? Is it Pager's, or Neuralink's?
Moving a cursor on a screen is one thing, but what if the neural activity encoded is more sensitive than that? What kind of rules around privacy exist to protect the user? These are all questions that need to be considered.
The rapid rise in interest in neurotechnology has also meant regulation has been slow to keep up. Because the way products are marketed informs the regulations they need to comply with, there is a fear that companies are sidestepping critical checks42 by marketing their neurotechnologies as "wellness" products rather than medical devices.
Conclusion
The field of neurotechnology encompasses many techniques and types of technology. From being able to record the activity of a single neuron firing, to modulating the activity of entire brain regions, there's no doubt neurotechnology is and will continue to change the way we treat neurological and psychiatric conditions.
As Florien Solzbacher of Blackrock Neurotech put it, "I do foresee that in 20-30 years, these types of implants will be just as common and acceptable as cardiac pacemakers are today."43 If Solzbacher's predictions come true, it will change the game for sufferers of dementia, mood disorders and neurodegenerative diseases. Neurotechnology has the potential to help us diminish the symptoms of these diseases, but also to augment the human experience. We just have to be open to it.
References
1. Regalado A. Brain implants could be the next computer mouse. MIT Technology Review. https://www.technologyreview.com/2021/10/27/1036821/brain-computer-interface-implant-mouse/ Published 2020. Accessed 7 January 2022.
2. Angeli C, Boakye M, Morton R et al. Recovery of Over-Ground Walking after Chronic Motor Complete Spinal Cord Injury. New England Journal of Medicine. 2018;379(13):1244-1250. doi:10.1056/nejmoa1803588
3. Müller O, Rotter S. Neurotechnology: Current Developments and Ethical Issues. Front. Syst. Neurosci. 2017;11:93. doi:10.3389/fnsys.2017.00093
4. Dibner B. Luigi Galvani | Italian physician and physicist. Encyclopedia Britannica. https://www.britannica.com/biography/Luigi-Galvani. Published 2021. Accessed January 6, 2022.
5. Drew L. Of Currents and Photons: Should Neuroscientists Use Imaging or Electrophysiology to Monitor Neural Activity?. Neuroscience from Technology Networks. https://www.technologynetworks.com/neuroscience/articles/of-currents-and-photons-should-neuroscientists-use-imaging-or-electrophysiology-to-monitor-neural-317794. Published 2019. Accessed January 6, 2022.
6. Ibid
7. Tozer A. Electrophysiology Goes Digital. Neuroscience from Technology Networks. https://www.technologynetworks.com/neuroscience/blog/electrophysiology-goes-digital-310617. Published 2018. Accessed January 6, 2022.
8. Electroencephalography (EEG). Brainsigns. https://www.brainsigns.com/en/science/s2/technologies/eeg. Published 2018. Accessed January 24, 2022.
9. Sirven J. Encyclopedia Of The Neurological Sciences. 2nd ed. Academic Press; 2014:1080-83.
10. Bazzigaluppi P. From Twitching Frogs to Brain Implants: 5 Key Technologies in Electrophysiology. Neuroscience from Technology Networks. https://www.technologynetworks.com/neuroscience/lists/from-twitching-frogs-to-brain-implants-5-key-technologies-in-electrophysiology-328001. Published 2019. Accessed January 7, 2022.
11. Brown A, Johnson B, Goodman M. Making Patch-pipettes and Sharp Electrodes with a Programmable Puller. J Vis Exp. 2008;20(939). doi:10.3791/939
12. Carter M, Shieh J. Guide To Research Techniques In Neuroscience. Chapter 3 - Electrophysiological Techniques. Academic Press; 2010:73-90. doi:10.1016/B978-0-12-374849-2.00003-3
13. Drew L. Of Currents and Photons: Should Neuroscientists Use Imaging or Electrophysiology to Monitor Neural Activity?. Neuroscience from Technology Networks. https://www.technologynetworks.com/neuroscience/articles/of-currents-and-photons-should-neuroscientists-use-imaging-or-electrophysiology-to-monitor-neural-317794. Published 2019. Accessed January 6, 2022.
14. Deep brain stimulation - Mayo Clinic. Mayoclinic.org. https://www.mayoclinic.org/tests-procedures/deep-brain-stimulation/about/pac-20384562. Accessed January 8, 2022.
15. Suchorska B, Ruge M. Deep brain stimulation: current applications and future prospects. Discov Med. 2015;20(112):403-11. PMID: 26760984
16. Ibid
17. Perlmutter J. and Mink J.Deep Brain Stimulation. Annu Rev Neurosci, 2006(29):229-257. doi: 10.1146/annurev.neuro.29.051605.112824
18. Rehncrona S. Johnels B. Widner H. Törnqvist A. Hariz M. and Sydow O. Long-term efficacy of thalamic deep brain stimulation for tremor: double-blind assessments. Mov Discord, 2003(18)2:163-70. doi: 10.1002/mds.10309
19. Perlmutter J. and Mink J.Deep Brain Stimulation. Annu Rev Neurosci, 2006(29):229-257. doi: 10.1146/annurev.neuro.29.051605.112824
20. Chail A, Saini R, Bhat P, Srivastava K, Chauhan V. Transcranial magnetic stimulation: A review of its evolution and current applications. Ind Psychiatry J. 2018;27(2):172-80. doi: 10.4103/ipj.ipj_88_18
21. Mackenzie R. Stimulation Sensation: The Non-Invasive Technologies Shaking Up Neuroscience. Neuroscience from Technology Networks. https://www.technologynetworks.com/neuroscience/articles/stimulation-sensation-the-non-invasive-technologies-shaking-up-neuroscience-346368. Published 2021. Accessed January 8, 2022.
22. Transcranial magnetic stimulation - Mayo Clinic. Mayoclinic.org. https://www.mayoclinic.org/tests-procedures/transcranial-magnetic-stimulation/about/pac-20384625. Accessed January 8, 2022.
23. Thair H, Holloway A, Newport R, Smith A. Transcranial Direct Current Stimulation (tDCS): A Beginner's Guide for Design and Implementation. Front Neurosci. 2017;(11):641. doi:10.3389/fnins.2017.00641
24. Allenby C, Falcone M, Bernardo L et al. Transcranial Direct Current Brain Stimulation Decreases Impulsivity in ADHD. Brain Stimul. 2018;11(5):974-81. doi:10.1016/j.brs.2018.04.016
25. Nitsche M, Cohen L, Wassermann E. Transcranial direct current stimulation: State of the art 2008. Brain Stimul. 2008;1(3):206-23. doi:10.1016/j.brs.2008.06.004
26. Filmer H, Dux P, Mattingley J. Applications of transcranial direct current stimulation for understanding brain function. Trends in Neuroscience. 2014;37(12):742-53. doi:10.1016/j.tins.2014.08.003
27. Coffman B, Clark V, Parasuraman R. Battery powered thought: Enhancement of attention, learning, and memory in healthy adults using transcranial direct current stimulation. Neuroimage. 2014;85(3):895-908. doi:10.1016/j.neuroimage.2013.07.083
28. Yu K, Niu X, Krook-Magnusson E, He B. Intrinsic functional neuron-type selectivity of transcranial focused ultrasound neuromodulation. Nat Commun. 2021;12(2519). doi:10.1038/s41467-021-22743-7
29. Forget Brain Surgery: Ultrasound Therapies Could Help Treat the Brain. Neuroscience from Technology Networks. https://www.technologynetworks.com/neuroscience/news/forget-brain-surgery-ultrasound-therapies-could-help-treat-the-brain-348503. Published 2021. Accessed January 8, 2022.
30. Essential Tremor. Focused Ultrasound Foundation. https://www.fusfoundation.org/diseases-and-conditions/neurological/essential-tremor. Published 2021. Accessed January 17, 2022.
31. Non-invasive focused ultrasound treatment (FUS) at Sheba Medical Center. Sheba Medical Center. https://www.shebaonline.org/treatment/fus/. Published 2020. Accessed January 17, 2022.
32. Shih J, Krusienski D, Wolpaw J. Brain-Computer Interfaces in Medicine. Mayo Clin Proc. 2012;87(3):268-79. doi:10.1016/j.mayocp.2011.12.008
33. Agashe H, Paek A, Zhang Y, Contreras-Vidal J. Global cortical activity predicts shape of hand during grasping. Front Neurosci. 2015;9(121). doi:10.3389/fnins.2015.00121
34. Olivieri E, Barresi G, Mattos L. BCI-based user training in surgical robotics. Annu Int Conf IEEE Eng Med Biol Soc. 2015:4918-21. doi:10.1109/EMBC.2015.7319495
35. Monkey Mindpong. YouTube.com: Neuralink; https://www.youtube.com/watch?v=rsCul1sp4hQ Published 2021. Accessed January 6, 2022.
36. Schirmann F. “The wondrous eyes of a new technology”—a history of the early electroencephalography (EEG) of psychopathy, delinquency, and immorality. Frontiers in Human Neuroscience. 2014;8. Accessed February 11, 2022. https://www.frontiersin.org/article/10.3389/fnhum.2014.00232
37. Mackenzie R. How Can We Make It Easier To Implant a Brain-Computer Interface?. Neuroscience from Technology Networks. https://www.technologynetworks.com/neuroscience/blog/how-can-we-make-it-easier-to-implant-a-brain-computer-interface-352026. Published 2021. Accessed January 9, 2022.
38.Hitti N. Elon Musk unveils updated Neuralink brain implant design and surgical robot. Dezeen. https://www.dezeen.com/2020/09/02/neuralink-elon-musk-brain-implant-technology/. Published 2020. Accessed January 9, 2022.
39. Telford T. Elon Musk wants to get inside your head. Literally. washingtonpost.com. https://www.washingtonpost.com/business/2019/07/17/elon-musk-wants-get-inside-your-head-literally/. Published 2019. Accessed January 9, 2022.
40. Mackenzie R. How Can We Make It Easier To Implant a Brain-Computer Interface?. Neuroscience from Technology Networks. https://www.technologynetworks.com/neuroscience/blog/how-can-we-make-it-easier-to-implant-a-brain-computer-interface-352026. Published 2021. Accessed January 9, 2022.
41. Mackenzie R. How Could a “Breakthrough” Brain-Computer Interface Help Patients?. Neuroscience from Technology Networks. https://www.technologynetworks.com/neuroscience/blog/how-could-a-breakthrough-brain-computer-interface-help-patients-356896. Published 2021. Accessed January 8, 2022.
42. Mackenzie R. Privacy in the Brain: The Ethics of Neurotechnology. Neuroscience from Technology Networks. https://www.technologynetworks.com/neuroscience/articles/privacy-in-the-brain-the-ethics-of-neurotechnology-353075. Published 2021. Accessed January 9, 2022.
43. Mackenzie R. How Can We Make It Easier To Implant a Brain-Computer Interface?. Neuroscience from Technology Networks. https://www.technologynetworks.com/neuroscience/blog/how-can-we-make-it-easier-to-implant-a-brain-computer-interface-352026. Published 2021. Accessed January 9, 2022.
About the author: Julia is a location-independent writer with a passion for communicating scientific ideas to the public. She holds a BSc (Hons) in Medical Science and a MSc in Sustainable Agriculture, and loves writing about neuroscience, behaviour, agriculture, ecology, conservation, and more. In her free time she loves dancing, hiking, and making music.