Freezing Life: The Current Trends in Cryopreservation
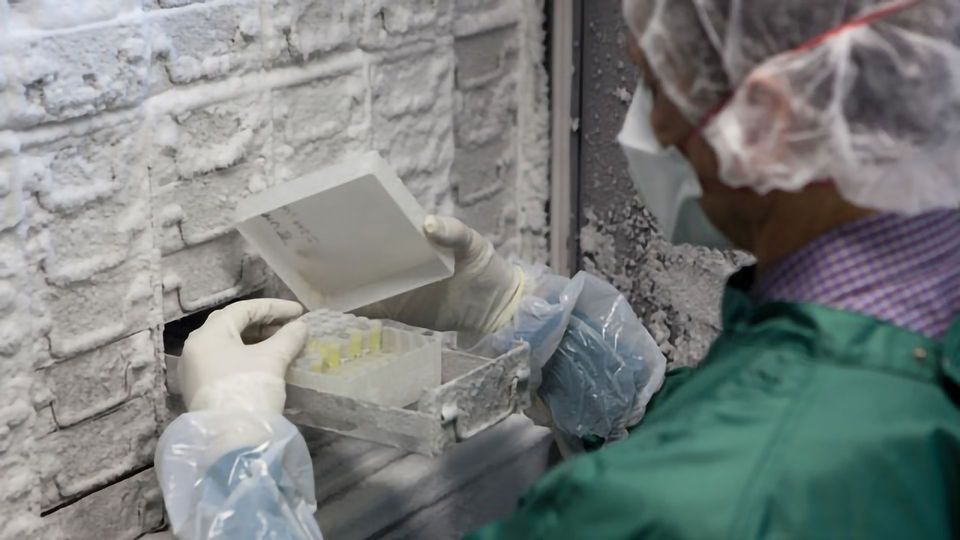
Complete the form below to unlock access to ALL audio articles.
Cryopreservation has become an indispensable step in the daily routine of scientific research as well as in a number of medical applications, ranging from assisted reproduction and transplantations to cell-based therapies and biomarker identification. It is hardly possible to picture today’s scientific and medical advancements without this technique.
The successful development and implementation of all the therapeutic and scientific discoveries involving cryopreservation relies on the correct and safe translation of the method from the laboratory to the clinical and manufacturing scale.
With the need to correctly use this technique, more research is focusing on optimizing cryopreservation methods and investigating what the long-term effects and consequences are on the physiology of the cryopreserved material.
The physics behind cryopreservation
In order to modify or troubleshoot a protocol, we should first understand how it works.
Cryobiology relies on very complex physico-chemical processes and reactions, but briefly, with the drop in temperature the water from the extracellular space transitions from liquid to solid state and with this ice crystal formation begins. With water being engaged into crystals the solute concentration increases and induces the osmotic efflux from the inside of the cells. The controlled reduction of temperature is needed to ensure the sufficient osmotic pressure difference that reduces the water levels inside the cell and thus prevents the formation of ice crystals within it. Simultaneously to water exit, cryoprotectant agent enters the cell and increases its viscosity, thus preventing the cell’s shrinkage due to dehydration1,2.
Even if correctly and successfully performed, all these events have their negative effects on the cells. Lipid membranes can be destructed by ice crystals or by temperature drop, dehydration causes structural deformation of the cell and cryoprotectants have cytotoxic effects. Ultimately these result in decreased post-thaw viability and proliferation, but it is also likely that they provoke deeper and complex disturbances in the cell’s identity and functionality.
Success of cell-based therapies relies on good cryopreservation practice
Cryopreservation by slow freezing is the golden standard in cell culture preservation. Although it is a largely used technique in basic research, the rise of cell-based therapies is bringing many questions on how accurately this procedure is being performed and how many factors should be considered when it needs to comply with medical or manufacturing standards.
An important part of cell therapy research is focused on adult stem cells (ASCs). ASCs can be derived from different sources such as peripheral blood, bone marrow or adipose tissue and display strong promises because of their capacity to differentiate into any cell type of the human body.
In recent work3, the team of Michael Pepper at the Institute for Cellular and Molecular Medicine in Pretoria, South Africa, explored the effects of cryopreservation on the differentiation ability of adipose tissue-derived stem cells (ADSCs). After analyzing gene expression of key adipogenic genes and the degree of differentiating cells, characterized with high levels of CD36 and intracellular lipid droplets, the scientists reported that slow freeze cryopreservation of cells shortly after their isolation causes no alterations on their ability to differentiate. Pepper is convinced of the necessity to perform such analysis when cryopreserving important cell pools: “It is critical to do a post-thaw analysis of cell function to determine how the cryopreservation may have affected the cells.”
His team is analyzing the effects of cryopreservation on other cell types largely used in cell-based therapies such as hematological stem cells and peripheral blood mononuclear cells (PBMCs). Although they didn’t observe major alterations in terms of immunophenotyping or the post-thaw proliferation of the cells, Pepper expresses his concern that more subtle characteristics might be affected.
Correct cryopreservation of cells intended for therapeutic use is crucial. “This is very important particularly as cells may persist for a long time in the recipient. This area of cell therapy research definitely requires more attention,” Pepper says. Moreover, his words reflect on the need to evaluate not only the direct post-thaw recovery, but to look deeper into the late-onset effects cryopreservation might have and ensure that transplanted cells have preserved their therapeutic properties.
Cryopreservation Guide - the basics of cellular cryopreservation for research & clinical use

Cryopreservation has become a routine practice in biomedical research and clinical medicine. When frozen and kept properly, specimens may remain in a state of suspended cellular metabolism indefinitely and can be thawed as needed. To reap the benefits of this process, it is important to have a thorough understanding of the key aspects of cryopreservation. Download this guide to the basics of cellular cryopreservation for research and clinical use.
Sponsored Content
Vitrification – the newcomer in tissue preservation
The other largely used cryopreservation technique is vitrification. The choice between the two methods depends on the properties of the material. Vitrification is mainly used for preservation of larger samples such as oocytes, embryos and tissues.
In contrast to slow freezing, vitrification relies on the fast freezing of the material by putting it in high concentration of cryoprotectant and in contact with liquid nitrogen. This method allows the direct transition of water from liquid to solid state without crystal formation. The highly concentrated cryoprotectant prevents ice formation and therefore there is no need for slow cooling.
Although vitrification has a great potential, there are a couple of parameters that are a point of concern. The quick and drastic freeze is possible thanks to the high concentration of cryoprotectant, but the latter is also associated with higher toxicity. In some cases, an additional limitation is the direct contact of the sample with liquid nitrogen which is a predisposition for viral or bacterial contamination.
The team of Christiani Amorim at the Institute for Experimental and Clinical Research in Louvain, Belgium, is approaching the challenges of vitrification in the context of ovarian auto-transplantation. Ovarian auto-transplantation consists of preserving a piece of ovarian tissue with active follicles from the pre-therapeutic ovary of a cancer patient, as chemotherapy often has damaging effects on the reproductive organs. This tissue sample will be conserved and auto-transplanted onto the patient’s ovary when she has recovered and wishes to become pregnant.
In their recent research4, the authors used stepped vitrification, in which the concentration of the cryoprotectant is gradually increased while simultaneously temperature decreases. This avoids ice crystal formation and also prevents cryoprotectant toxicity.
Although stepped vitrification has previously given good results in bovine ovarian tissue5, this was not the case for human ovarian tissue. The scientists didn’t detect normal follicles following thawing and linked this to high cryoprotectant toxicity. Indeed, they observed all signs of dimethyl sulfoxide (DMSO)-related cell membrane damage: significant organelle damage, cell membrane disintegration and apoptosis. These observations imply on the variability of outcomes that the method could give when applied to the same type of tissue but from a different organism.
Amorim is positive about the future of their method and recognizes the need for further research on the topic: “I can see a great potential in the stepped vitrification approach, but I also believe that there is a lot we still need to learn before thinking about using it as method of choice for human ovarian tissue cryopreservation. The high cryoprotectant concentration that should be applied in this approach is my first concern. (…) Our study clearly showed that 50% DMSO is too high, so we need to try lower concentrations or combine it with other cryoprotectants.”
Are the most critical points the most neglected ones?
Although broadly and daily used, the method of freezing life for a certain period of time followed by its subsequent revival, relies on complex principles of physics that are not as broadly understood and considered. “Most scientist who use the techniques are likely to have a limited understanding of the physics of cryopreservation,” says Pepper. Amorim is on the same page when it comes to this matter: “I think most part of us, scientists, do not grasp the complexity involved in such procedure.”
This overall limited understanding leads to the fact that often the most critical points in the protocol are the most neglected ones. It could appear that the most challenging part of cryopreservation is the long-term storage of the materials at ultra-low temperatures, in reality it is not. Type and concentration of cryoprotectant agents as well as the time to freeze down and the number of cells play much more critical roles according to Pepper. As well as, Amorim reminds us, the thawing steps, which are often disregarded.
Future perspectives
If there is one thing we can be certain about, it is that cryopreservation is here to stay and will be indispensable for the future progress of scientific research and development of therapeutic solutions. The question we need to resolve is how to evaluate and prevent the negative effects cryopreservation has on the biological material. “Cryopreservation requires optimization, and standardization of optimized techniques would allow us to compare apples to apples and to remove a potentially confounding variable in interpreting data. An automated approach based on validated parameters would assist in this regard,” Pepper is convinced.
Amorim envisions cryopreservation development in the same direction: “I think that the first step is to have a robust procedure and then develop a cryopreservation machine that can handle several samples simultaneously.” And also implies on the importance of further assessment of the consequences of cryopreservation not only on vitality, but at a much deeper level: “Once we find a protocol that can preserve the follicle pool, we need to ensure that the final cryoprotectant concentration does not interfere with epigenetic mechanisms, and particularly genomic imprinting.”
It will be interesting to see how the need for accuracy and upscaling will modulate this long-used technique and what further understanding of its effects it will bring.
Professor Michael Pepper and Professor Christiani Amorim spoke to Maya Chergova, PhD for Technology Networks. Professor Michael Pepper is an expert in the field of cell-based therapies and Director of the Institute for Cellular and Molecular Medicine at the University of Pretoria, South Africa. Professor Christiani Amorim is Professor at the Institute for Experimental and Clinical Research at the Catholic University of Louvain, Belgium. She has extensive experience in the development of ovarian and follicular cryopreservation and transplantation methods.
References
- Woods, E. J., Thirumala, S., Badhe-Buchanan, S. S., Clarke, D. & Mathew, A. J. (2016). Off the shelf cellular therapeutics: Factors to consider during cryopreservation and storage of human cells for clinical use. Cytotherapy 18, 697–711.
- Bakhach, J. (2009). The cryopreservation of composite tissues: Principles and recent advancement on cryopreservation of different type of tissues. Organogenesis 5, 119–126.
- Durandt, C., Dessels, C., da Silva, C., Murdoch, C. & Pepper, M. S. (2019). The Effect of Early Rounds of ex vivo Expansion and Cryopreservation on the Adipogenic Differentiation Capacity of Adipose-Derived Stromal/Stem Cells. Sci. Rep. 9, 1–13.
- Leonel, E. C. R. et al. (2019). Stepped vitrification technique for human ovarian tissue cryopreservation. Sci. Rep. 9, 1–12.
- Corral, A. et al. (2018). Ovarian tissue cryopreservation by stepped vitrification and monitored by X-ray computed tomography. Cryobiology, 81, 17–26.