An Introduction to Electroporation – A Tool for Transfection and Competent Cell Generation
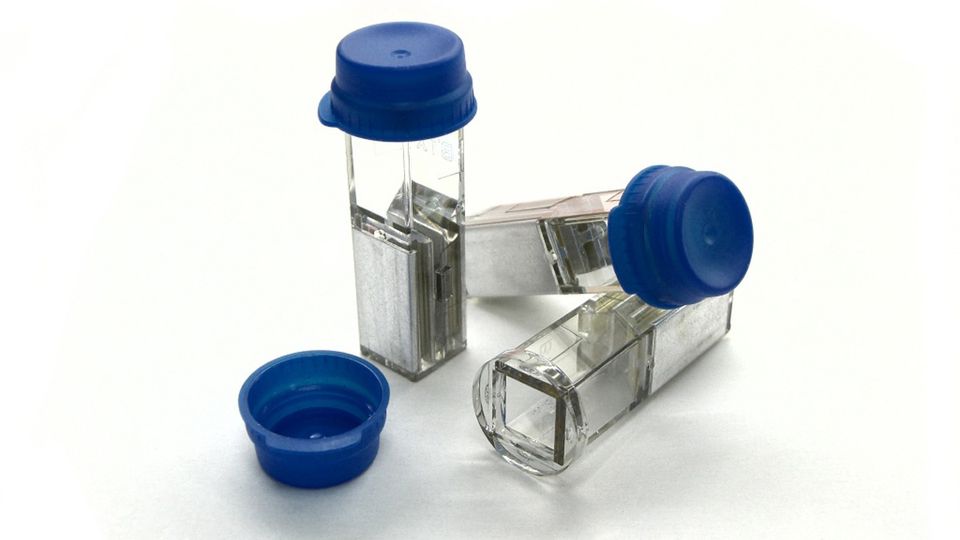
Complete the form below to unlock access to ALL audio articles.
There are numerous reasons that you may want to deliver something into a cell, be it genetic material in the form of a plasmid or maybe a therapeutic drug. However, in order to do that, you first have to get it through the cell membrane without doing permanent harm to the cell – a delicate process and one for which electroporation is a vital tool. In this article, we will consider what electroporation is, how it works and how it’s used.
What is electroporation?
What is electroporation?
Electroporation, also called electropermeabilization, is an efficient, non-viral delivery system that allows genetic material (DNA and RNA), proteins, drugs or other molecules to enter cells. It uses an accurately pulsed electrical current to create temporary pores in the cell membrane through which the molecules can then pass. This process can be used on a wide variety of cells including mammalian,1 insect,2 yeast,3 plant4 and bacterial cells.5
Once inside, drugs and other molecules may act on the cell. Genetic material may remain independent (as a plasmid) or become integrated into the host genome depending on the downstream experimental steps. While electroporation, first described in 19726 but not named until 1982,7 has been used as an in vitro tool for many years, in 1991, the first in vivo electroporation8 was used to deliver genetic material and it is now being investigated for use to deliver gene and cell therapies for a range of conditions. Since then, techniques have also been developed to deliver electroporation in utero in mice9 and other experimental animals and in ovo in chickens and snakes.10
How does electroporation work? The electroporation machine and electroporation cuvettes
When a cell is able to take up free DNA or RNA, it is termed “competent”. Some cell types or microbial species, such as Bacillus subtilis,11 are naturally competent and they can be induced to uptake DNA simply by switching nutrient concentration to induce stress. Many cells, however, are not naturally competent or may only uptake certain material, such as antimicrobial resistance cassettes, under specific conditions that are complex to reproduce in the laboratory. Chemicals can be used to induce competence in some species, such as Escherichia coli (E. coli),12 but again, this does not work for all cell types or microbial species. Instead, the process of electroporation can make non-competent cells competent and amenable to experimental manipulation.
For in vitro electroporation, a suspension of target cells is mixed in a conductive solution with the molecule you wish to introduce into the cells and placed in a cuvette (Figure 1). Electroporation cuvettes have metal plates on either side of the sample chamber that allow an electrical current to be passed through the mixture. The cuvette is placed into a chamber of the electroporator that has corresponding electrical contacts, enabling the cuvette to form an electrical circuit. Controls on the electroporation machine allow the user to set the voltage, waveform and duration of the electrical pulse to be delivered, which should be optimized to the target cell type. An electrical pulse is then passed through the sample chamber.
Figure 1: A diagram of the main components of an electroporator with cuvette loaded. Credit: Richard Wheeler, reproduced under the GNU Free Documentation license.
The electrical pulse disturbs the phospholipid bilayer of the cell membrane,13 causing pores to be formed (Figure 2). This occurs asymmetrically with pores forming first on the anode side of the cell. At the same time, the electrical potential across the membrane is increased. This drives charged molecules, such as DNA, to adsorb to the membrane, first on the cathode side of the cell, and move through these pores and into the cell14 (Figure 3). The cell wall, present in plant cells and some bacteria, is a major barrier for the movement of macromolecules into cells. Consequently, protoplasts (cells from which the cell wall has been removed) are typically used for electroporation,4, 15 although methods for electroporation of cells with the wall intact have been developed.16
Figure 2: Schematic diagram showing disruption of the cell membrane and pore formation during electroporation.
After the electrical pulse has passed, the pores begin to reseal.17 The rate18 at which this occurs varies from cell to cell but is typically in the region of milliseconds to minutes. At this stage, cells are delicate and must be treated carefully until they have had a chance to divide.
Figure 3: Schematic diagram showing the steps and associated charges during electroporation that lead to the introduction of exogenous material into the cell, in this case a plasmid.
Example electroporation protocol
When establishing an electroporation protocol, it is important to choose parameters19 that enable permeabilization while at the same time minimizing disturbance to the cell membrane to maximize cell viability, experimental reproducibility and efficiency. Factors to consider include the:
- waveform
- pulse time
- field strength
Pulses are generally either classed as square wave or exponential decay wave. Square wave pulses rise rapidly to their set voltage, maintain the level and then swiftly cut off at the pulse end. With exponential decay waves on the other hand, the voltage rises rapidly to the target value and is then allowed to decline over time. Square waves are typically used for mammalian cells, while exponential decay waves are more often used for bacterial, yeast, insect and plant cells as well as some types of mammalian cell. While transfection is possible with both waveforms in the differing cell types, transformation efficiencies tend to be low using square waves in bacterial, yeast and insect cells and exponential decay waves tend to reduce the viability of many mammalian cells.19
The duration of the pulse (normally micro- to milliseconds) can be inputted directly for square waves. However, with exponential decay waves, this is not a predetermined value but is impacted by other experimental conditions due to the nature of the wave. Instead, the pulse length is referred to in terms of the “time constant” (TC) and is the length of time it takes for the electrical pulse to decay to a third of its original voltage (Figure 4). Efficiency is typically optimal around a TC of 5.0–5.1 for cells like E.coli, with values of 4.0–5.0 considered fairly normal. However, efficiency reduces the further from this value it goes. Lower time constants can be caused by issues like poor quality DNA, insufficient washing or having too dense a sample of cells. Higher time constants can indicate that the pores have been open for too long and will likely result in higher cell mortality.
Figure 4: Graph showing the reduction in voltage of an exponential decay wave with the time constant (T) indicated where the voltage reaches a third of its initial value.
If the pulse length is increased, the voltage is typically decreased and vice versa to help prevent irreparable cell damage.
The field strength is the voltage delivered across the electrode gap and is measured in kV/cm. Cuvettes are available with differing gap sizes, so it is important to take this into consideration when optimizing your voltage. If the necessary field strength is known, it is possible to calculate the voltage required for any given cuvette size using the following equation:
Other factors that can impact the field strength required for success include cell size (with smaller cells typically requiring higher voltages for a given electrode gap) and temperature (with higher voltages generally required at cooler temperatures).
In contrast to bacterial electroporation, which generally works best with short, high voltage pulses (hundreds to thousands of volts for micro- to milliseconds), electroporating cells or tissue from higher organisms often requires milder conditions.20 Many electroporators offer a selection of preset programs for specific cell types that have already been optimized.
While for many cell types a single pulse is sufficient, some cell types require two or more pulses, typically delivered automatically by a multi-electroporator at preset intervals.
Let’s consider an example protocol for bacterial electroporation.
- Grow a healthy culture of cells. For bacterial strains, mid-exponential phase cultures that are actively dividing are a good rule of thumb. For some cells, such as bacterial strains that produce large amounts of hyaluronic acid capsule, additional enzymes may need to be added to the growth media to remove the capsule to improve the process efficiency.
- Cells need to be free of salts as otherwise the current will pass around them in the solution when the electrical pulse is delivered. Therefore, wash the cells to remove salts; ice cold 0.5 M sucrose can be a good option. When pelleting the cells to remove the growth media and washes, treat them gently and use a chilled centrifuge to minimize cell damage. Once washed, keep them on ice and gently resuspend in the same sucrose solution.
- As well as chilling the wash and cells, place the cuvette and substance to be introduced on ice to keep things as cold as possible.
- Pipette the washed cells into the cuvette and gently add the substance to be introduced, pipetting gently to mix.
- Before placing the cuvette in the electroporator, ensure it is thoroughly dried. Even a few drops of water on the outside can lead to an electrical short circuit that will spoil your experiment (and produce a loud bang!).
- Deliver the electrical pulse and check the time constant is within the expected parameters.
- Add ice cold media to the cells and allow them to warm back up to their normal growth temperature in the incubator. Try to prevent sudden shocks as this can increase cell death and reduce the efficiency of your experiment.
- Once they are warm and actively dividing again (which will depend on the cell type or strain being cultured), cells can be cultured as normal. At this point, selective agents to promote growth of cells that have taken up the target substance over those that have not can be introduced if they are being used.
While for many cell lines, cooler experimental temperatures can improve cell survival and experimental success, there are some cells for which this in not the case.21, 22 It is therefore important to optimize any protocols to suit your specific cells.
While electroporation has traditionally been performed on cells in suspension, it is now also possible to perform electroporation on adherent cells.23 This can be very helpful when working with cells in culture where it may be desirable to avoid trypsinization.
Electroporation transformation - Making bacterial competent cells with an electroporator
There are numerous reasons why it may be desirable to manipulate the genetic makeup of a bacterial cell. For example, to:
- Create an engineered strain of a bacterium for a vaccine
- Elucidate gene function
- Insert a plasmid encoding a tag to allow cells to be tracked
- Engineer in genes encoding a desirable product in order to use them as mini protein factories
A key part of many bacterial genetic engineering protocols, however, relies on the delivery of the modified genetic material into the bacterial cells. For strains that are not naturally competent, electroporation makes this possible.24 DNA, often in the form of a plasmid, can then pass into the cells. In bacteria, this process is known as transformation. A means of selecting for cells that have successfully been transformed is often incorporated into these plasmids too, such as an antibiotic resistance gene. Once the cells have been allowed to recover from electroporation, they may then be cultured in the presence of the selective agent so those that have not successfully taken up the target DNA are killed off while those that have survive and replicate. Cells that contain the target plasmid are called transformants. Depending on the downstream applications, the plasmid may remain independent, or steps may then subsequently be taken to integrate the plasmid into the bacterial genome.
Electroporation transfection – Electroporation for eukaryotic cells
While in bacteria, the process of introducing nucleic acids into a cell is termed transformation, in eukaryotic cells this is termed transfection25 and as with bacteria can be achieved using electroporation. As well as allowing scientists to engineer the eukaryotic cells themselves26 or introduce other molecules, transfection also acts as a means to engineer viruses27 to help understand their pathogenesis, biology28 and to create modified strains for vaccination purposes.
As cells themselves, bacteria can be electroporated and engineered directly, however, the process of genetic engineering is different for viruses. Here, host cells, such as mammalian or insect cells, are infected with the target virus and it is these host cells that undergo electroporation to introduce the desired genetic material to then be incorporated during viral replication.
Other uses of electroporation
In vivo gene therapy
As with many genetic manipulation techniques, gene therapies29 require a means to deliver the additional, modified or “fixed” genetic code to their target cells or inactivate a problematic gene. There are a number of ways of doing this that are being investigated, including using viral gene transfer, nanoparticles and electroporation, for the treatment of conditions including inherited retinal dystrophies.30
Irreversible electroporation
While the electroporation we have discussed here is deemed to be reversible electroporation and the membrane pores formed are transient, irreversible electroporation has also been used in cancer treatment31 as a technique for the ablation of solid tumors.32 Like reversible electroporation, a current is applied to the cells, causing pores to appear in the membrane. However, in this case the aim is to upset the cell homeostasis, resulting in tumor cell death. There is also evidence to suggest that the release of intracellular tumor antigens acts as an in situ “tumor vaccine”, offering a promising immunomodulatory therapeutic aspect too.33
In utero electroporation
As a tool to study the development process, the ability to deliver plasmid DNA, for example encoding marker proteins,34 to specific cells in a developing fetus has attracted significant attention, especially from the neuroscience community (ref 2).35,36 First performed successfully in 2001,9 in utero electroporation has been used to answer questions about dendrite growth and synaptic connectivity34 and cell fate and migration during development.37 So far, it has yet to be used to deliver gene therapy to human fetuses.
Electroporation vaccine and drug delivery
While electroporation is typically associated with delivery of genetic material to a cell, it can also be used to enable drug and vaccine38 delivery. This provides access for large or hydrophobic substances that are otherwise unable to cross the skin effectively.39 It has been used for dermal and transdermal drug delivery40,41 for conditions including cancer.42 DNA vaccines43 against anthrax, plague,44 human papillomavirus (HPV)45 and SARS-CoV-2,46 among others, have also been developed that utilize this mode of delivery as most mammalian cells are not naturally competent and direct injection of naked DNA has had limited success.
Common problems with electroporation
- Electroporation parameters - Getting the right electroporation conditions47 can be tricky and requires optimization when working with a new cell line or strain. If the conditions are too harsh, the cells may be permanently damaged, reducing cell viability and transformation/transfection rate. Too mild and it may not create effective pores, also reducing success rates.
- Buffer conditions – The best buffer47 for your electroporation will vary depending on your target cells and should be optimized.
- Quantities of cells and plasmid/component to be introduced into cells – It is important that appropriate and balanced quantities of cells and the exogenous material to be introduced into the cells are used. Overly high cell densities can lead to arcing while too few cells will reduce experimental success. Too little plasmid/component for introduction into the cells compared to the number of cells present will also reduce the potential achievable efficiency. Again, this is something that will need to be optimized.
- Quality of cells – If the cells being used in the experiment are not in good condition at the start, this will negatively impact experimental success. Try to ensure they are healthy, free of contaminants and actively dividing to improve recovery post-electroporation. Cells of a high passage number may work less efficiently so should be avoided if possible. Overly vigorous pipetting can also damage the cells.
- Quality of nucleotides/components for insertion – Poor quality or contaminated nucleotide samples will reduce transformation/transfection efficiency and may reduce cell survival. High salt levels and large plasmids (over 10 kb) may also reduce experimental efficiency so should be considered when planning experiments.
- Care of cells – Cells are delicate following electroporation and must be treated with care during recovery. Failure to do so will increase cell death.
- Arcing – Keeping components on ice is an excellent way to keep them cool and preserve your cells during the electroporation procedure. However, failure to completely dry the cuvette before placing it in the chamber will likely cause a short circuit, leading to experimental failure and potentially damaging the equipment. As well as moisture in the chamber, residual salt, too high a cell density or bubbles, for example from overly vigorous pipetting, may also cause arcing to occur in the cuvette chamber. Ensure salts are removed thoroughly, cell densities are optimized – check the manufacturer’s recommendations for guidance – and when adding and mixing the components, do so gently. This will help to prevent the introduction of bubbles and reduce damage to the cells.
References
1. Chicaybam L, Barcelos C, Peixoto B, et al. An efficient electroporation protocol for the genetic modification of mammalian cells. Front. Bioeng. Biotechnol. 2017;4. doi:10.3389/fbioe.2016.00099
2. Mann SG, King LA. Efficient transfection of insect cells with baculovirus DNA using electroporation. J Gen Virol. 1989;70 (Pt 12):3501-3505. doi:10.1099/0022-1317-70-12-3501
3. Benatuil L, Perez JM, Belk J, Hsieh CM. An improved yeast transformation method for the generation of very large human antibody libraries. Protein Eng. Des. Sel. 2010;23(4):155-159. doi:10.1093/protein/gzq002
4. Bates GW. Chapter 26 Electroporation of plant protoplasts and tissues. In: Galbraith DW, Bourque DP, Bohnert HJ, eds. Methods in Cell Biology. Vol 50. Methods in Plant Cell Biology. Academic Press; 1995:363-373. doi:10.1016/S0091-679X(08)61043-2
5. Chassy BM, Mercenier A, Flickinger J. Transformation of bacteria by electroporation. Trends Biotechnol. 1988;6(12):303-309. doi:10.1016/0167-7799(88)90025-X
6. Neumann E, Rosenheck K. Permeability changes induced by electric impulses in vesicular membranes. J Membrain Biol. 1972;10(1):279-290. doi:10.1007/BF01867861
7. Neumann E, Schaefer-Ridder M, Wang Y, Hofschneider PH. Gene transfer into mouse lyoma cells by electroporation in high electric fields. EMBO J. 1982;1(7):841-845. doi:10.1002/j.1460-2075.1982.tb01257.x
8. Titomirov AV, Sukharev S, Kistanova E. In vivo electroporation and stable transformation of skin cells of newborn mice by plasmid DNA. Biochim Biophys Acta - Gene Struc Exp. 1991;1088(1):131-134. doi:10.1016/0167-4781(91)90162-F
9. Saito T, Nakatsuji N. Efficient Gene transfer into the embryonic mouse brain using in vivo electroporation. Dev. Biol. 2001;240(1):237-246. doi:10.1006/dbio.2001.0439
10. Cárdenas A, Borrell V. A protocol for in ovo electroporation of chicken and snake embryos to study forebrain development. STAR Protoc. 2021;2(3):100692. doi:10.1016/j.xpro.2021.100692
11. Rahmer R, Morabbi Heravi K, Altenbuchner J. Construction of a super-competent Bacillus subtilis 168 using the PmtlA-comKS inducible cassette. Front. Microbiol. 2015;6. doi:10.3389/fmicb.2015.01431
12. Green R, Rogers EJ. Chemical transformation of E. coli. Methods Enzymol. 2013;529:329-336. doi:10.1016/B978-0-12-418687-3.00028-8
13. Tieleman DP. The molecular basis of electroporation. BMC Biochem. 2004;5(1):10. doi:10.1186/1471-2091-5-10
14. Kandušer M, Miklavčič D. Electroporation in biological cell and tissue: An overview. In: Electrotechnologies for Extraction from Food Plants and Biomaterials. Food Engineering Series. Springer; 2008:1-37. doi:10.1007/978-0-387-79374-0_1
15. Bates GW. Plant transformation via protoplast electroporation. In: Hall RD, ed. Plant Cell Culture Protocols. Methods In Molecular BiologyTM. Humana Press; 1999:359-366. doi:10.1385/1-59259-583-9:359
16. Furuhata Y, Sakai A, Murakami T, et al. A method using electroporation for the protein delivery of Cre recombinase into cultured Arabidopsis cells with an intact cell wall. Sci Rep. 2019;9(1):2163. doi:10.1038/s41598-018-38119-9
17. Saulis G. Pore disappearance in a cell after electroporation: theoretical simulation and comparison with experiments. Biophys J. 1997;73(3):1299-1309. doi:10.1016/S0006-3495(97)78163-3
18. Saulis G, Venslauskas MS, Naktinis J. Kinetics of pore resealing in cell membranes after electroporation. J Electroanal. Chem. Interfacial Electrochem. 1991;321(1):1-13. doi:10.1016/0022-0728(91)85564-6
19. Jordan ET, Collins M, Terefe J, Ugozzoli L, Rubio T. Optimizing electroporation conditions in primary and other difficult-to-transfect cells. J Biomol Tech. 2008;19(5):328-334. PMC2628074
20. Transfection of mammalian cells by electroporation. Nat Methods. 2006;3(1):67-68. doi:10.1038/nmeth0106-67
21. Tu Q, Yin J, Fu J, et al. Room temperature electrocompetent bacterial cells improve DNA transformation and recombineering efficiency. Sci Rep. 2016;6(1):24648. doi:10.1038/srep24648
22. Wards BJ, Collins DM. Electroporation at elevated temperatures substantially improves transformation efficiency of slow-growing mycobacteria. FEMS Microbiol. Lett. 1996;145(1):101-105. doi:10.1111/j.1574-6968.1996.tb08563.x
23. Raptis L, Vultur A, Brownell HL, et al. Electroporation of adherent cells in situ for the Study of signal transduction and gap junctional communication. In: Li S, ed. Electroporation Protocols: Preclinical and Clinical Gene Medicine. Methods in Molecular BiologyTM. Humana Press; 2008:173-189. doi:10.1007/978-1-59745-194-9_12
24. Chassy BM, Mercenier A, Flickinger J. Transformation of bacteria by electroporation. Trends Biotechnol. 1988;6(12):303-309. doi:10.1016/0167-7799(88)90025-X
25. Potter H, Heller R. Transfection by electroporation. Curr Protoc Mol Biol. 2003;CHAPTER:Unit-9.3. doi:10.1002/0471142727.mb0903s62
26. Chicaybam L, Barcelos C, Peixoto B, et al. An efficient electroporation protocol for the genetic modification of mammalian cells. Front. Bioeng. Biotechnol. 2017;4. doi:10.3389/fbioe.2016.00099
27. Russell TA, Stefanovic T, Tscharke DC. Engineering herpes simplex viruses by infection-transfection methods including recombination site targeting by CRISPR/Cas9 nucleases. J Virol Methods. 2015;213:18-25. doi:10.1016/j.jviromet.2014.11.009
28. Hoffmann E, Neumann G, Kawaoka Y, Hobom G, Webster RG. A DNA transfection system for generation of influenza A virus from eight plasmids. PNAS. 2000;97(11):6108-6113. doi:10.1073/pnas.100133697
29. Prud’homme GJ, Glinka Y, Khan AS, Draghia-Akli R. Electroporation-enhanced nonviral gene transfer for the prevention or treatment of immunological, endocrine and neoplastic diseases. Curr Gene Ther. 2006;6(2):243-273. doi:10.2174/156652306776359504
30. Ziccardi L, Cordeddu V, Gaddini L, et al. Gene therapy in retinal dystrophies. Int J Mol Sci. 2019;20(22):5722. doi:10.3390/ijms20225722
31. Miller L, Leor J, Rubinsky B. Cancer cells ablation with irreversible electroporation. Technol Cancer Res Treat. 2005;4(6):699-705. doi:10.1177/153303460500400615
32. Ruarus AH, Vroomen LGPH, Geboers B, et al. Percutaneous irreversible electroporation in locally advanced and recurrent pancreatic cancer (PANFIRE-2): A multicenter, prospective, single-arm, Phase II study. Radiology. 2020;294(1):212-220. doi:10.1148/radiol.2019191109
33. Zhang N, Li Z, Han X, et al. Irreversible electroporation: An emerging immunomodulatory therapy on solid tumors. Front. Immunol. 2022;12. doi:10.3389/fimmu.2021.811726
34. Zhang L, Getz SA, Bordey A. Dual in utero electroporation in mice to manipulate two specific neuronal populations in the developing cortex. Front. Bioeng. Biotechnol. 2022;9. doi:10.3389/fbioe.2021.814638
35. Yamashiro K, Ikegaya Y, Matsumoto N. In utero electroporation for manipulation of specific neuronal populations. Membranes. 2022;12(5):513. doi:10.3390/membranes12050513
36. Saito T. Chapter three - Embryonic in vivo electroporation in the mouse. In: Wassarman PM, Soriano PM, eds. Methods in Enzymology. Vol 477. Guide to Techniques in Mouse Development, Part B: Mouse Molecular Genetics, 2nd Edition. Academic Press; 2010:37-50. doi:10.1016/S0076-6879(10)77003-8
37. Rosin JM, Kurrasch DM. In utero electroporation induces cell death and alters embryonic microglia morphology and expression signatures in the developing hypothalamus. J Neuroinflammation. 2018;15(1):181. doi:10.1186/s12974-018-1213-6
38. Keane-Myers AM, Bell M. Evolution of electroporated DNA vaccines. In: Li S, Cutrera J, Heller R, Teissie J, eds. Electroporation Protocols: Preclinical and Clinical Gene Medicine. Methods in Molecular Biology. Springer; 2014:269-278. doi:10.1007/978-1-4614-9632-8_24
39. Prausnitz MR, Bose VG, Langer R, Weaver JC. Electroporation of mammalian skin: a mechanism to enhance transdermal drug delivery. Proc Natl Acad Sci U S A. 1993;90(22):10504-10508. doi:10.1073/pnas.90.22.10504
40. Medi BM, Layek B, Singh J. Electroporation for dermal and transdermal drug delivery. In: Dragicevic N, I. Maibach H, eds. Percutaneous Penetration Enhancers Physical Methods in Penetration Enhancement. Springer; 2017:105-122. doi:10.1007/978-3-662-53273-7_7
41. Yarmush ML, Golberg A, Serša G, Kotnik T, Miklavčič D. Electroporation-based technologies for medicine: Principles, applications, and challenges. Annu. Rev. Biomed. Eng. 2014. 16:295–320 doi:10.1146/annurev-bioeng-071813-104622
42. Łapińska Z, Szwedowicz U, Choromańska A, Saczko J. Electroporation and electrochemotherapy in gynecological and breast cancer treatment. Molecules. 2022;27(8):2476. doi:10.3390/molecules27082476
43. Chiarella P, Fazio VM, Signori E. DNA Vaccination by Electrogene Transfer. IntechOpen; 2011. doi:10.5772/17648
44. Albrecht MT, Livingston BD, Pesce JT, Bell MG, Hannaman D, Keane-Myers AM. Electroporation of a multivalent DNA vaccine cocktail elicits a protective immune response against anthrax and plague. Vaccine. 2012;30(32):4872-4883. doi:10.1016/j.vaccine.2012.04.078
45. Sun Y, Peng S, Qiu J, et al. Intravaginal HPV DNA vaccination with electroporation induces local CD8+ T-cell immune responses and antitumor effects against cervicovaginal tumors. Gene Ther. 2015;22(7):528-535. doi:10.1038/gt.2015.17
46. Smith TRF, Patel A, Ramos S, et al. Immunogenicity of a DNA vaccine candidate for COVID-19. Nat Commun. 2020;11:2601. doi:10.1038/s41467-020-16505-0
47. Sherba JJ, Hogquist S, Lin H, Shan JW, Shreiber DI, Zahn JD. The effects of electroporation buffer composition on cell viability and electro-transfection efficiency. Sci Rep. 2020;10(1):3053. doi:10.1038/s41598-020-59790-x