5 Influential Neuroscientists, Past and Present
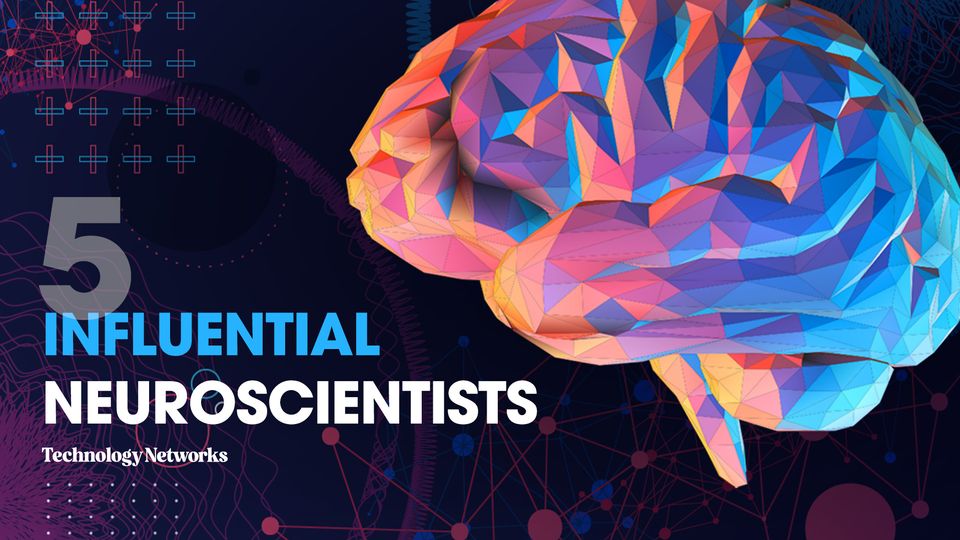
While there are now end of year ranking lists for just about everything, from cheese (a Spanish goat’s cheese called Olavidia triumphed in 2021) to words (this year’s winner (sigh) – non-fungible token, or NFT) science is a little harder to measure up. While the Nobel Prize tries, and sometimes fails, to be an authority on important scientific achievement, comparing innovations like AlphaFold to the development of a COVID-19 vaccine is a fairly fruitless task.
Instead, this year we have decided to pick five inspiring neuroscientists from the last hundred or so years, in no particular order, and tell you why their influence on other researchers will likely endure well into the future of science.
Brenda Milner
In case you doubted the caliber of academic we are discussing in this list, let’s start with Brenda Milner, who was born while World War I was raging and yet was still supervising researchers one hundred years later. Milner, who studied at Newnham College in Cambridge before emigrating to Canada in 1944, was a pioneering neuropsychologist. While Milner initially taught psychology at the Université de Montreal, discoveries across a host of brain systems, including learning, memory and intelligence that she made while at the Montreal Neurological Institute and Hospital have since cemented her legendary legacy.
Most famously, Milner worked with Henry Molaison, known until his death in 2008 as simply H.M., an epilepsy patient who, after undergoing drastic surgery to cure his rampant seizures, lost the ability to form new memories. While Molaison’s memory for events and facts was stuck in 1953, a year before his surgery, Milner’s experiments showed that he was still able to learn new motor skills. This discovery revealed the existence of more than one type of memory, a finding that has had incalculable impact on the field of cognitive neuroscience.
Milner’s deductions about how brain systems were mapped onto structures, decades before neuroimaging could reveal detailed pictures of the brain, were revolutionary. These included findings on how people with damage to their frontal lobes perform behavioral tasks and how the brain’s two hemispheres individually contribute to learning and memory.
Milner’s influence has not just been through these groundbreaking experiments, but via her close and careful work with trainee neuroscientists and as an (unintended) icon of female neuroscientists from an era where men dominated the field. Having recently celebrated her 103rd birthday, Milner continues to engage in research work.
Ben Barres
Ben Barres, who died at the end of 2017 aged 63, was hugely influential in our understanding of how glial cells contribute to the brain. Despite glia making up nine-tenths of the brain’s cells, research largely viewed the cells as inconsequential padding for bringing together more important neurons – the word glia comes from an ancient Greek word meaning “glue”.
Barres and his lab helped reframe glia, which are now recognized as playing a crucial role in hundreds of brain processes:
- Myelin produced by glial cells called oligodendrocytes plays a role in information processing
- Deficits in scavenging microglial immune cells may influence Alzheimer’s disease pathology
- Glial cells could be a target for intervention in Huntington’s disease
Barres’ relentless focus on glia, summarized by William Newsome, director of the Stanford Neurosciences Institute as a “big career bet on the possibility that there was gold in glia,” helped bring attention to these cells, revealing how glia could regulate synapse production and provide trophic support to neurons.
Barres was a pioneer in more ways than one. He was the first openly transgender member of the National Academy of Sciences and fought a long and hard battle to disprove toxic biases about gender and ability in science that persist even today. Barries transitioned in 1997, when the unique pressures and discriminations faced by the trans community were not part of public discourse.
At the time, Barres, suffering under the weight of gender dysphoria, wrestled with what he saw as a binary choice: transition and potentially end his career or continue to live under his assigned sex and potentially commit suicide. Barres ultimately found that, as a man in science, all the cards were stacked in his favor. That his immediate response was to put himself in the thick of what he described as a “street fight” for gender equality in science shows why his early death was such a significant loss to neuroscience.
Terje Lømo
In 1964, one of the most significant discoveries in modern neuroscience began with a chance meeting in a street in Oslo. Navy doctor Terje Lømo, who was on leave to look for a job, bumped into University of Oslo professor Per Andersen, who was looking for a new member of his lab team. Shortly after, Lømo began his PhD in Andersen’s lab.
Lømo’s resulting doctoral work ultimately led to the discovery of long-term potentiation (LTP), the strengthening of synaptic connections between neurons that can ultimately produce long-lasting increases in signal transmission. LTP has come to be recognized as essential to the processes of learning and memory in the brain.
Lømo examined the electrical signals produced by stimulation of a nerve pathway in the hippocampus called the perforant path. Brief, high-frequency bursts of targeted stimulation, Lømo noticed, resulted in a rapid and enduring increase in the strength of synaptic connections. While he published these findings in 1966, it was not until 1968 when British scientist Tim Bliss joined the Anderson lab, that Lømo’s research would take the next step into neuroscience legend. The pair would collaborate for several years over the late 1960s and early 1970s, eventually producing a landmark paper that expanded on Lømo’s initial findings – the theory of LTP was born.
The idea of LTP and its opposite, long-term depression (LTD) – where synaptic transmission efficacy is reduced, have been greatly expanded on over the last fifty years. But Lømo’s early work, which gave a mechanistic explanation to Donald Hebb’s postulate – “cells that fire together, wire together” – has remained one of the most significant discoveries in cellular neuroscience.
Karl Friston
Karl Friston, a British polymath, has the kind of academic track record that suggests he could make a pretty chunky contribution to any sphere of human progress, given enough time and thought. Neuroscience is lucky enough that he started out his career as a psychiatrist, where his work with patients at Littlemore Hospital imparted a deep curiosity for the altered way in which people with schizophrenia, depression or psychosis saw the world.This led to pioneering mathematical research in the 1990s that developed a technique called statistical parametric mapping (SPM). This set of processes allows brain imaging techniques like functional magnetic resonance imaging to infer patterns of activity from brain scans. Without SPM, modern brain imaging wouldn’t be possible. While this innovation alone would probably allow Friston a place in neuroscience history, his tireless work ethic has produced a body of work with nearly 300,000 citations. Recent publications and conference papers include efforts to model SARS-CoV-2’s spread, explorations of the brain networks active during dreaming and a look at how statistical techniques could predict the Earth’s resilience against climate change.
In fact, his work on brain imaging was merely an aperitif ahead of the main effort of Friston’s work – the free-energy principle. This theory is a gargantuan, notoriously complex approach to understanding how individual agents, be they bodies, organs or cells, interact with the world. It is as ambitious in scale as Newtonian theories of thermodynamics, and Friston believes it could advance fields as diverse as psychiatry, physics and artificial intelligence. As systems based on the free-energy principle concept like active inference and surprise become more common, Friston’s greatest impacts on how we understand the brain could be yet to come.
Beth Stevens
Science isn’t known for being a rapid process. Efforts to advance science proceed at a glacial pace, and the recognition of those advances as being significant can take decades. This makes identifying research from the 21st century that is already influencing and likely to continue influencing neuroscience a difficult proposition. Nevertheless, the work of Harvard Medical School professor Beth Stevens undoubtedly points the way to the future of neuroscience.Stevens’ work builds upon that of aforementioned glial pioneer Ben Barres, in whose lab she began postdoctoral research in 2004. Her focus has been in establishing a role for immune glial cells in the pruning of synapses. This pruning process begins early in development. The infant brain is stuffed full of neurons – the human brain begins with 100 million nerve cells, 15% more than will be found in an adult brain. The task of whittling down this excessive cell count to mature brain levels falls to immune cells in the brain called microglia, that hungrily gobble up excess synaptic connections and neurons.
Stevens’ lab identified an unexpected role for immune proteins, called the complement system, in tagging up cells for this microglial mukbang. Since then, including in papers coauthored with Barres, Stevens has established herself as a leader in microglial neurobiology. The influence of this research is just beginning to be felt. Microglia have now been identified as potential players and therapeutics in brain diseases at both the beginning and end of life, such as autism and Alzheimer’s disease.
Honored in 2012 by President Obama with the Presidential Early Career Award for Scientists and Engineers, Stevens exemplifies how influence in science builds and is built from the work of other researchers. Discoveries made by previous generations echo down to provide scaffolds and buttresses to provide ever-more advanced research with a strong foundation. The scientists on our list will surely continue to influence researchers for generations to come.